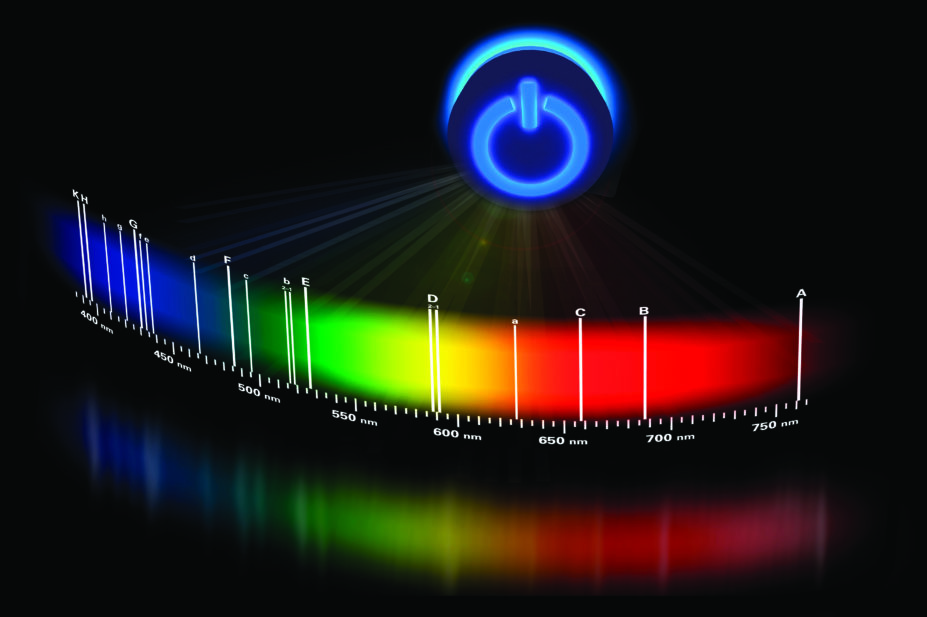
Carlos Clarivan / Science Photo Library / Shutterstock.com
We use the lightbulb to signify a new idea, but in the nascent field of photopharmacology, light itself is the new idea. With some clever chemistry, drug molecules are being designed that change their shape and activity with the flick of a light switch. Using these ‘photoswitches’, it is possible to limit medicine activity to the parts of the body where it is needed, offering side effect-free therapies for conditions from diabetes to cancer.
Chemist Dirk Trauner, currently professor of chemistry at New York University, was one of the earliest pioneers of photopharmacology: “I was initially interested in what is now called optogenetics,” he says, referring to the field that has developed a method to control brain function in mice using genetically engineered neurons and light. In 2003, advances in optogenetics led Trauner, then at the University of California, Berkeley, to the idea of developing drugs containing synthetic light-switching molecules, and the field of photopharmacology was born.
Source: Christopher Then
Chemist Dirk Trauner, who is currently at New York University, was one of the earliest pioneers of photopharmacology
Azobenzenes — the photoswitches that could
Trauner started his research by looking for a molecule that had two mirror image versions — or isomers — which could be switched using light.
The isomers needed to have vastly different shapes, so the switch was likely to also alter the conformation of any attached drug and therefore the drug’s ability to reach its biological target. This way light could be used to switch drug activity on or off. The obvious molecule was azobenzene (C12H10N2), which was discovered over 150 years ago and is used extensively as a dye and food colourant. The molecule comprises two benzene rings linked by a nitrogen — nitrogen double bond (see figure 1).
Referring to azobenzenes, Trauner says: “I always call them ‘the little photoswitches that could’ — very reliable little engines with which to drive things.” The more stable trans isomer has the benzene rings on opposite sides but, on irradiation with ultraviolet (UV) light, this switches to the cis confirmation, with the benzene rings sitting next to each other. In time, the molecule reverts to the more stable trans form. Azobenzenes also benefit from a large pre-existing catalogue of toxicity data owing to their extensive industrial use.
Figure 1: Azobenzene
Azobenzene has two isomers that can be switched by using light
Restoring sight
The most advanced photopharmaceutical research and development is being carried out by California-based company Photoswitch Biosciences. Set up in 2008 by a team including Trauner and Berkeley neuroscientists Richard Kramer and Ehud Isacoff, the company is developing a light-responsive drug that could restore sight.
Jay Trautman, chief executive officer at Photoswitch Biosciences, says the company is on the threshold of starting clinical trials. “We have reasonably encouraging data at this point with a new formulation of the compound that leads us to believe that we will likely be able to select the candidate in the next couple of months,” he says. The lead compound could be in clinical trials by 2018, he adds.
Andrew Blatz, chief scientific officer at Photoswitch Biosciences, says that the company expects to formulate a treatment administered by monthly injection.
Loss of photoreceptor cells is the leading cause of blindness in the developed world and is largely irreversible. It causes degenerative conditions such as age-related macular degeneration (AMD), as well as genetic diseases, such as retinitis pigmentosa. With this in mind, Trauner and his colleagues realised that the light-sensitive receptor proteins within the eye, called opsins, are essentially in-built photoswitches. Found in the membranes of retinal neurons, they work together with the light-sensitive vitamin A-based molecule retinal. A photon of light results in the photoisomerisation of retinal, which induces a conformational change in the opsin protein. This causes a cascade of cell signalling that results in an electrical signal being sent to the brain.
Having discovered this, Trauner and his team at Berkeley decided to try to replace the function of opsins using a drug containing a switchable azobenzene molecule. The idea was to find a molecule that could directly block potassium ion channels in the membranes of retinal cells, and in doing so, set off a signalling cascade similar to that created when photoreceptor proteins are working.
The first successful candidate, published in 2012[1]
, was the molecule diethylamine-azobenzene-quaternary ammonium (DENAQ), which replicates the light switching function of opsins, blocking the cell potassium-ion channels when activated by light and unblocking the channels in the dark.
In trials on blind mice, DENAQ restored light sensitivity as shown by increased levels of movement and learning abilities. More recently, in 2016, Trauner’s group has developed another class of photochromic ligands based on the amino acid AMPA (α-amino-3-hydroxy-5-methyl-4-isoxazolepropionic acid), but modified to include a photoswitchable side chain[2]
.
Obviously, restoring some light sensitivity in mice is a long way from restoring meaningful sight in humans, but one finding is making the team at Photoswitch Biosciences hopeful.
“The retina has many different kinds of cells, some which fire for example when a light is turned on, some that fire when a light is turned off and are responsive to changes in the direction of a light,” explains Blatz. “We normally process all of this information in a very complicated way, so when you are firing any given neuron in a retinal regeneration situation it’s difficult to say exactly how this would be conceived [by the brain].” However, the photoswitchable drug seems to preferentially target ion channels in retinal ganglion cells, a subset of neurons on the inner surface of the retina that would normally deliver a neural pulse to the brain when light is turned off. “This gives us a lot of hope that what the patient would experience would be more interpretable than if random neurons were firing,” adds Blatz.
Chemotherapy side effects
Trauner’s most recent breakthrough, in July 2016, is the synthesis of a photoswitchable chemotherapy drug based on combretastatin A-4 (CA4), a compound discovered 20 years ago in bushwillow tree bark. The molecule has the ability to stop cell division by inhibiting the production of microtubules — rigid, hollow rods of tubulin polymers that make up a cell’s cytoskeleton.
Drugs that inhibit the production of microtubules make up a major class of chemotherapies but, as with many cancer drugs, their powerful effects lead to severe side effects, which can limit dosages.
Trauner believes photopharmacology may offer a solution. “We have developed a class of drugs that only hits microtubules when you also irradiate,” he explains. The original CA4 molecule has a trimethoxybenzene ring, giving cis and trans isomers of the drug. The cis isomer is more potent than the trans isomer. By replacing a carbon double bond with a nitrogen double bond and adding some additional functional groups, Trauner created a new set of compounds that he named photostatins, whose drug activity can be controlled by blue light. The light causes a switch to the cis conformation, which is 250 times more toxic to cancer cells than the trans isomer[3]
.
In tests on worm embryos, Trauner’s laboratory were able to achieve targeted single cell death, with neighbouring untargeted cells continuing to divide unharmed. “We are quite confident that this is going to translate into a therapy down the road, where you provide a drug in an inactive non-toxic form and then locally unleash its toxicity,” Trauner says.
We are quite confident that this is going to translate into a therapy down the road, where you provide a drug in an inactive non-toxic form and then locally unleash its toxicity
This approach could be an ideal treatment for skin cancers, but how might the therapy be used for tumours inside the body? Trauner proposes internal light would be feasible using tiny cell-sized implanted wireless LEDs. “They are already in development and they will be clinically ready for use in a few years — maybe even biodegradable ones,” he says.
Diabetes and beyond
Trauner has also applied the photopharmacology approach to diabetes drugs. Type 2 diabetes is now estimated to affect about 10% of Europe’s population, with 4 million sufferers in the UK. It is commonly treated with drugs based on sulfonylurea, which stimulates insulin secretion by binding to receptors on pancreas beta-cells. But treatment comes with some serious side effects, such as blood sugar crashes and increases in cardiovascular disease. Limiting drug release to the pancreas might prevent these events by restricting the drug’s actions to where it’s needed, therefore reducing off-target physiological effects.
Working alongside diabetes researcher David Hodson from Imperial College London, Trauner tested a new compound based on the third generation sulfonylurea drug, glimethoride, but containing an azobenzene unit[4]
. The drug would need to be activated after a meal, Hodson explains, most likely using an implanted blue LED: “The drug switches on very quickly in milliseconds, and because it switches off within about two seconds you are able to get quite fine control.”
The drug switches on very quickly in milliseconds, and because it switches off within about two seconds you are able to get quite fine control
He adds that so far tests in animals have been successful. “We have given the drug to rats and mice to control their glucose level in vivo and we can do that quite nicely.”
Photopharmacology could also be used to tackle antibiotic resistance. Chemistry Nobel Prize (2016) winner Ben Feringa, whose group at the University of Groningen in the Netherlands has been at the forefront of research in the field, has decades of experience in designing molecular switches for nanoelectronics and saw an opportunity to apply some of that experience to the problem of antibiotic resistance.
If antibiotic activity could be switched off outside the human body, this would help to prevent resistance developing. Feringa and his colleague Wiktor Szymanski, also from the University of Groningen, have designed several switchable versions of a quinolone antibiotic containing an azobenzene unit. These could be switched on to their active state with UV light but switched off automatically within hours[5]
.
Source: Courtesy of Wiktor Szymanski
Wiktor Szymanski, from the University of Groningen, has designed several switchable versions of a quinolone antibiotic containing an azobenzene unit
The phototherapeutic window
The idea of photoswitchable drugs has captured the interest of some of today’s most talented synthetic chemists, but it remains to be seen whether photopharmacology is clinically practical. The first issue is the ease and safety of penetrating the body with light (see figure 2). Azobenzene switches at a wavelength of 340nm, within the UV range, but we know that UV light can damage cells.
Figure 2: Penetrating the body with light
The ease and safety with which light penetrates the body depends on the wavelength of the light used. Azobenzene molecules switch at a wavelength of 340nm, within the ultraviolet (UV) range, but UV light is known to be damaging to cells. Researchers are trying to find azobenzenes that switch at higher wavelengths, in the 700—750nm range, so less damaging light can be used to activate the drug.
A) UV light is known to cause damage to cells
B) Azobenzene molecules switch at 340nm
C) Penetration of light <700nm is limited by absorption by haemoglobin
D) Light with a wavelength of 700-750nm can penetrate deep into the tissues
E) Penetration of light >900nm is limited by absorption by water
Szymanski is now based at the radiology department of the University Medical Centre Groningen and is learning from medical colleagues who currently deliver photodynamic therapies. “These guys had been struggling with the question of delivering light for many years before we came to the game and one of the things that we learnt from them is the phototherapeutic window,” he says.
These guys had been struggling with the question of delivering light for many years before we came to the game and one of the things that we learnt from them is the phototherapeutic window
In photodynamic therapy, injected photosensitising drugs are irradiated, releasing free radical oxygen species that kill cancer cells. The technique requires the light used to be within the 700–900nm wavelength range. “On the one hand these are relatively low energy photons compared to UV photons, which means that they are not that damaging to the tissue.” Szymanski explains that light above or below these boundaries is likely to be absorbed by molecules in the surrounding tissue and may never reach its intended target. “Anything more energetic [lower wavelength] will be very quickly absorbed by haemoglobin. On the upper side, at the border of 900nm, we start to be limited by the absorption of water, which doesn’t absorb strongly but we have a lot of it.”
This has meant a push to find azobenzenes that switch at higher wavelengths. If you are able to operate in the 700–750nm range you can go deep into tissues, so “the redder the better”, explains Trauner. One of the first people to achieve this, in 2011, was Andrew Woolley from the University of Toronto. He noted that azobenzenes that switched at higher wavelengths had been synthesised in the past, but they switched back within microseconds. “We managed to find ways to modify the molecule and slow that process down, so you shine light on it and it doesn’t go back so quickly,” explains Woolley. “It’s mostly by making substitutions [on the benzene rings], so sterically congesting the molecule to help slow that process down. One we have got going now, goes back to the trans form a million times slower than it would otherwise[6]
.”
Using such clever design, the switches can also be manipulated so the molecule stays in the active state for anything from one second to hours.
Courtesy of Andrew Woolley
Andrew Woolley, from the University of Toronto, was one of the first people to synthesise an azobenzene that switches at a higher wavelength
Building on this, Feringa and Szymanski published work in 2016 on a simplified synthesis of red-light switchable azobenzenes[7]
, which makes the production of photopharmaceuticals a little more feasible. “This was the limiting factor for a long time,” says Szymnaski. But now the issue is being able to synthesise drugs with these new azobenzenes. “You have to have your cake and eat it — [you have to have] a red-light switchable azobenzene and still be able to make a drug based on it.”
You have to have your cake and eat it — [you have to have] a red-light switchable azobenzene and still be able to make a drug based on it
But, as the field grows, Szymanski says there will still be a need for more diverse types of photoswitches that are not based on azobenzenes. One type of molecule already being investigated is dithienylethene, which switches from a flexible, open-ring structure to a rigid, closed-ring isomer (see figure 3).
Figure 3: Dithienylethene
Dithienylethene is being investigated as a potential photoswitch
There are other practical considerations to address before photopharmaceuticals turn up on pharmacy shelves. For example, how acceptable would LED implants be to patients and doctors? And how effective will local treatments really be? Diseases like cancer generally need some systemic treatment because of the risk of metastases.
Getting biological
So far, most photopharmaceuticals have been designed based on small molecule drugs, but given that almost 30% of new drugs approved by the US Food and Drug Administration are now biologics, can the approach also work with peptides or protein drugs? Extending their work on diabetes drugs, Trauner and Hodson recently designed a photoswitchable version of an incretin mimetic — a synthetic peptide drug that acts like the glucose-lowering incretin hormones to stimulate insulin production[8]
.
Woolley has also been looking at coupling photoswitches to proteins. “I think that there is some potential there — certainly you can drive changes in the confirmation of these things.
“But,” he adds, “it’s a bit ‘hit and miss’, we have had some successes but it’s not easily predictable.” Woolley and others are now creating photoswitchable molecules that can probe basic cell biology.
Hodson says that for pharmaceutical companies, currently photopharmacology is having a bigger impact as a tool “to allow them to understand better the mechanisms involved in how receptors work”. This is becoming particularly useful for investigating ion channel proteins. Many disease states are caused by ion channel pathologies but development of drugs addressing these has been disappointing.
Current techniques are cumbersome and expensive but controlling ion channels using light is providing an alternative. In addition to their vision restoring therapy, the Photoswitch Biosciences team is planning to beta test an instrument for high-throughput ion channel drug discovery this year. It has generated cell lines expressing cells with photoswitchable ion channels for assaying drug molecule effects on cells. “We have made a prototype instrument that has proof of concept that this works and we are now on our way to manufacturing a second generation device,” says Trautman.
It is still too early to assess the prospects for photopharmacology. The field is still in its infancy, but it’s starting to grow up. The first international meeting for photopharmacology is being held on 16 February 2017 in Groningen. But at this stage, the industry is waiting to see how the field progresses. One concern is the cost of the two rounds of toxicity testing that would be required for a drug with two states.
In the meantime, the hunt goes on for new photoswitches and new technologies for irradiating these molecules inside the body. “[Delivering light] is going to be the ultimate challenge,” says Szymanski, but adds chemists also have a challenge to meet. “Molecular scientists have to be ready with the molecules that can respond to this light,” he says.
Szymanski believes the future for photopharmacology is in selectively targeted treatments with luminescent compounds, which is currently “a bit of a dream,” he says. But the idea would be to attach luminescent molecules to a disease-targeting system like an antibody in the presence of a photoswitchable drug. “I don’t know where the disease spot is, but the antibody does and with it you can start sending around photons and activating the chemotherapy around it.” Szymanski admits this offers up many more questions than it answers, but it is a scintillating look at just what might be possible in the future.
References
[1] Polosukhina A, Litt J, Tochitsky I et al. Photochemical restoration of visual responses in blind mice. Neuron 2012;75:271–282. doi: 10.1016/j.neuron.2012.05.022
[2] Laprell L, Hüll K, Stawski P et al. Restoring light sensitivity in blind retinae using a photochromic AMPA receptor agonist. ACS Chem Neurosci 2016;7:15–20. doi: 10.1021/acschemneuro.5b00234
[3] Borowiak M, Nahaboo W, Reynders M et al. Photoswitchable inhibitors of microtubule dynamics optically control mitosis and cell death. Cell 2016; 162:403–411. doi: 10.1016/j.cell.2015.06.049
[4] Broichhagen J, Schönberger M, Cork SC et al. Optical control of insulin release using a photoswitchable sulfonylurea. Nat Commun 2014; 5:5116. doi: 10.1038/ncomms6116
[5] Velema WA, van der Berg JP, Hansen MJ et al. Optical control of antibacterial activity. Nat Chem 2013;5:924–928. doi: 10.1038/nchem.1750
[6] Bahalhavaeji DM, Samanta A, Beharry S et al. Red-shifting azobenzene photoswitches for in vivo use. Acc Chem Res 2015; 48:2662-2670. doi: 10.1021/acs.accounts.5b00270
[7] Hansen MJ, Lerch MM, Szymanski W et al. Direct and versatile synthesis of red-shifted azobenzenes. Angew Chem Int Ed 2016;55:13514–13518. doi: 10.1002/anie.201607529
[8] Broichhagen J, Podewin T, Meyer-Berg H et al. Optical control of insulin secretion using an incretin switch. Angew Chem Int Ed 2015;54:15565–15569. doi: 10.1002/anie.201506384
You may also be interested in
The importance of diverse clinical imagery within health education
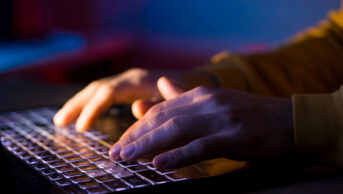
Government should consider ways to prevent ‘inappropriate overseas prescribing’ of hormone drugs, review recommends
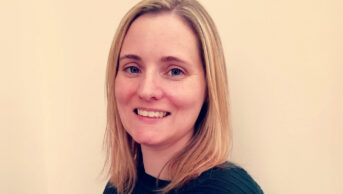