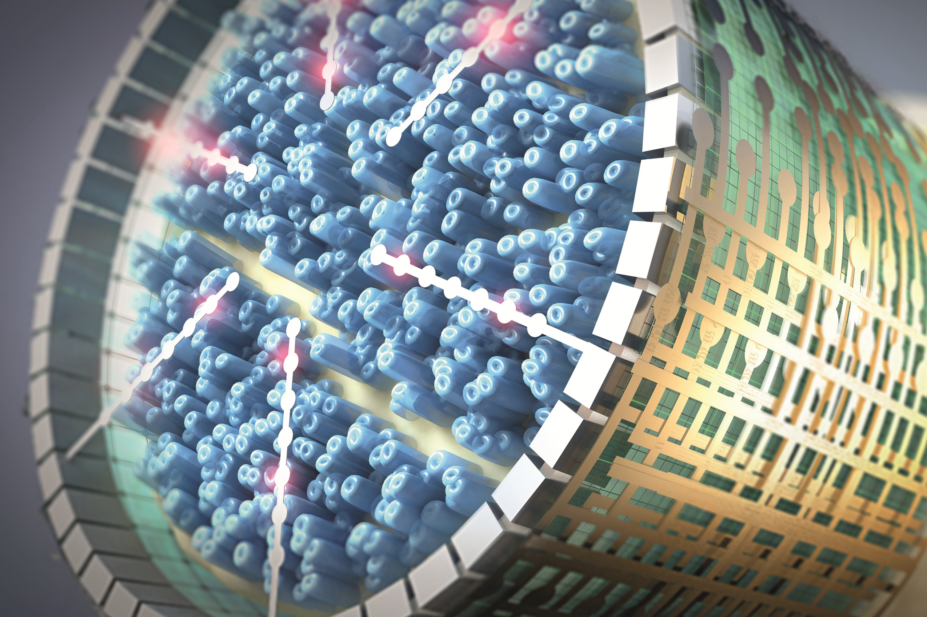
Courtesy of GSK
Imagine a prescription from your doctor, not for tablets but for a tiny electrical device implanted on a nerve in your neck. The device will monitor and treat your condition — whether it is diabetes, asthma, hypertension or even cancer — by modulating electrical impulses.
Pharmaceutical drugs can be highly effective, but don’t work for everyone. They tend to work systemically, often causing a variety of adverse effects, and rely on patient adherence. As a result, there are still countless chronic diseases that remain untreated or poorly treated by mainstream medicine. Enter bioelectronic medicines, a new group of therapies that work by transmitting electrical impulses along nerve fibres, rather than through molecular mechanisms. Tapping into the electrical wiring of the body, bioelectronic medicines — also called electroceuticals — could transform pharmaceutical treatment of many chronic diseases, providing an alternative or adjunct to traditional chemical or biological drugs. With technical advances and burgeoning research activity, this revolutionary approach to treating disease is starting to become a reality.
“By understanding mechanisms and interfacing electronics with the nervous system and other tissues, it may be possible to improve disease diagnosis and treatment, treat previously untreatable problems, and better prevent disease development,” says Peder Olofsson, a senior researcher at the Karolinska Institute in Sweden.
Source: Courtesy of Peder Olofsson
Peder Olofsson, a senior researcher at the Karolinska Institute in Sweden, says it is likely that more big pharmaceutical companies will diversify into bioelectronic medicine
Modulating electrical impulses
All the body’s organs are connected by peripheral nerves to the central nervous system, which influences their function via a pattern of electrical impulses (also known as action potentials) that run along the fibres (axons) in the nerve. Those patterns of electrical impulses are different when organs dysfunction in the case of chronic disease — for example, in the airways of healthy lungs compared with asthmatic lungs. The aim of bioelectronic medicine is to restore the ‘healthy’ pattern by implanting on individual nerves or specific bundles of axons tiny devices that can detect and selectively modulate (by stimulating or blocking) electrical impulses passing along them. The result should be accurate, continuous treatment of chronic disorders but with fewer side effects than typically encountered with conventional medicines.
“Drugs are based on exercising the chemical component of our nervous systems and tend to act very globally. Electroceuticals act locally,” explains Wouter Serdijn, a bioelectronics researcher at Delft University of Technology in the Netherlands and University College London. “Moreover, contrary to drugs, electroceuticals have an instantaneous effect and their effect is reversible. It takes quite some time for drugs to [exert] their beneficial effect and, as a consequence, it takes quite some time to be able to administer the right dose.”
Source: Courtesy of Wouter Serdijn
Wouter Serdijn, a bioelectronics researcher at Delft University of Technology in the Netherlands and University College London, says stimulating and recording nerves is a delicate and highly selective task
Stimulating neurons may release many neurotransmitters that may be of relevance — this would be very difficult to mimic by administering [drug] compounds exogenously
“An advantage of stimulating neurons is that it can activate an endogenous pathway used by the body in homeostatic conditions. This is a more natural approach to treating disease, compared to using drugs that may act on many receptors and places,” adds Guy Boeckxstaens, a neurogastroenterology researcher at the Translational Research Center for GI Disorders (TARGID) at University Hospital Leuven in Belgium. “Also, stimulating neurons may release many neurotransmitters that may be of relevance — this would be very difficult to mimic by administering [drug] compounds exogenously.”
“Bioelectronic medicines have the potential of doing to the pharmaceutical industry what biopharmaceuticals did to small molecules back in the 1980s,” says Imran Eba, partner at Cambridge, Massachusetts-based Action Potential Venture Capital (APVC), a bioelectronics fund backed by GlaxoSmithKline (GSK) aimed at invigorating this nascent field.
Customised personal medicine
Electrically stimulating parts of the body to alleviate illness has been used for decades, such as pacemakers for regulating heartbeat and cochlear implants to improve hearing. There are also neuromodulation tools such as spinal cord stimulators to treat pain, and deep brain stimulation for managing epilepsy or Parkinson’s disease. Although they can be effective, the devices used in these cases can sometimes be a little clumsy, delivering electrical pulses to relatively large areas or groups of nerve fibres, resulting in unwanted side effects.
The bioelectronic medicine approach of harnessing the body’s peripheral wiring takes the concept to a whole new level of precision and has broad potential applications: targeting the nerves that control bronchi constriction, for instance, could treat asthma, while controlling nerves in the stomach or pancreas could regulate food intake and insulin production to manage diabetes. Even nerves to ovaries or tumours could be targeted to treat infertility and cancer. Bioelectronic medicines may also allow patients and their physicians to monitor health in real time.
“An implant collecting neural signals can tell whether blood sugar levels are increasing or heart rate is decreasing. It can detect early signs of stroke before the incidence of stroke actually occurs,” says bioelectronics pioneer Kevin Tracey, president and chief executive officer of the Feinstein Institute for Medical Research, New York. “In other words, bioelectronic medicine has the potential to deliver highly customised personal medicine.”
The potential of modulating peripheral nerve signals to control organ function has not gone unnoticed by key funders of medical research. The US National Institutes of Health (NIH) announced a US$248m, seven-year SPARC (stimulating peripheral activity to relieve conditions) programme in 2014 to progress bioelectronic medicines, with particular focus on heart disease, asthma and gastrointestinal disorders. In October 2016, the first recipients of US$20m from this funding were named, marking the start of the core programme.
The US Defense Advanced Research Projects Agency (DARPA)’s is also funding research on bioelectronics medicines. DARPA’s ElectRx programme, which began in 2015, aims to establish a new biomedical, therapeutic capability using targeted stimulation of the peripheral nervous system.
GSK, the first large pharmaceutical company to act on the potential of action potentials, has been researching bioelectronic medicines since 2012. It has designated a US$1m innovation prize and a US$5m fund for bioelectronics research, invested US$50m in APVC, and is funding 33 research projects worldwide among academics and institutions. In August 2016, GSK, together with Verily Life Sciences (formerly Google Life Sciences), formed Galvani Bioelectronics, a new company dedicated to developing bioelectronic medicines. According to Eleanor Bunch, GSK’s director of global communications, GSK has established preclinical proof of concept in a small number of disease areas. Galvani’s initial focus will centre on establishing clinical proofs of principle in inflammatory, metabolic and endocrine disorders, including type 2 diabetes, and its first bioelectronic medicine should be ready for approval within the next seven years.
Mapping neural circuits
Research and development in the bioelectronic medicines sector is essentially divided into two sections. One half is focused on mapping action potential patterns in neural circuits to see how they differ in healthy and diseased conditions, and then trying to work out how to intervene electrically to change them without unwanted effects. The other is focused on the technical side, engineering miniaturised implantable sensors, modulators and power sources that are compatible with biological tissues.
It’s a tall order. “The problem with nerves is that they usually are grouped in bundles and they carry information to and from the brain, from and to the organ; often to more than one organ or to more than one part of an organ,” explains Serdijn. “So stimulation and recording nerves becomes a delicate and highly selective task.”
Challenges include understanding signalling and its exact effects (rather than trial and error), targeting specific nerve fibres rather than the entire bundle, the biocompatibility and longevity of the devices, as well as powering them and enabling closed-loop operation to adjust treatment to the therapeutic needs of the patient.
In terms of biological effect, a major research and development focus is on the vagus nerve, which comprises around 100,000 motor and sensory fibres innervating organs from the oesophagus and lungs to the intestines and heart. It controls involuntary functions, such as breathing, digestion and heart rate, and stimulating the vagus nerve has produced encouraging results in animal models in a range of diseases[1],[2],[3]
.
In July 2016, a breakthrough first-in-human study proved that electrical stimulation of part of the vagus nerve with an implantable bioelectronic device significantly reduced rheumatoid arthritis (RA) symptoms in several patients, some of whom had previously failed to respond to any other form of pharmaceutical treatment (including methotrexate, tumour necrosis factor [TNF] agonists and biologics), with no serious side effects[4]
. RA, a chronic inflammatory disease affecting around 400,000 people in Britain, causes stiff, swollen and painful joints. Stimulating part of the vagus nerve that leads to the spleen for one to four minutes daily inhibited production of TNF, an inflammatory molecule targeted by many pharmaceutical drugs.
“The clinical trial… was a watershed moment for the field of bioelectronic medicine,” says co-author Tracey. “It demonstrated conclusively that an electronic device targeting a basic mechanism we discovered in the laboratory, the inflammatory reflex, can be targeted in humans with RA.”
The promise of using bioelectronic devices targeting disease mechanisms has advanced closer to realisation
The inflammatory reflex is the automatic mechanism whereby the central nervous system, via the vagus nerve, regulates the immune response to infection, inflammation or injury[5]
.
Tracey says that since inflammation of this kind plays a crucial role in many diseases ranging from diabetes to cancer, “the promise of using bioelectronic devices targeting disease mechanisms has advanced closer to realisation”.
Initial results from another ongoing study, presented at the United European Gastroenterology Week in Vienna in October 2016, showed that a bioelectronic treatment for Crohn’s disease, which also targets the inflammatory reflex, significantly reduced symptoms in six out of eight patients with severe disease, and was well tolerated. Crohn’s disease is usually treated with steroids, immunosuppressants and surgery, which are risky and don’t work for everyone. The study data show a direct correlation between the bioelectronic treatment and suppression of pro-inflammatory cytokines, including TNF.
The treatment was developed by SetPoint Medical, a company co-founded by Tracey and involved in the RA study. “We do believe that SetPoint’s medical system platform may provide an alternative treatment option to many patients who suffer from chronic inflammatory diseases and are incomplete responders to conventional therapies, either owing to tolerability, efficacy, or usability difficulties,” says Raju Joshi, the company’s vice president of clinical affairs.
Figure 1: Neuromodulation for rheumatoid arthritis
Source: SetPoint
Electrical stimulation of the vagus nerve with an implantable bioelectronic device affects splenic T-cells and macrophages to reduce production of tumour necrosis factor (TNF), an inflammatory mediator, leading to reduced rheumatoid arthritis symptoms in some patients.
1) A microregulator stimulates the vagus nerve. The signals sent through the vagus and splenic nerve trigger a reduction in the activation of T cells and macrophages in the spleen
2) There is a reduced production of systemic inflammation mediators and reduced activation of circulating immune cells
3) This leads to decreased inflammation, joint damage and pain
Meanwhile, earlier stage research is under way elsewhere in a variety of indications. For example, Paul Frenette, director of the Albert Einstein College of Medicine, New York, and colleagues are exploring the role of the autonomic nervous system in prostate cancer formation and metastasis. “Our research is mostly mechanistic but we are also exploring this idea [of a bioelectronic treatment of prostate cancer],” he says.
Boeckxstaens and his colleagues at TARGID are investigating the extent to which stimulating the vagus nerve in the abdomen — not in the neck, like most other groups — can reduce intestinal inflammation. “We are looking at the mechanisms involved and how they can be applied to several GI-related diseases, including post-operative ileus, colitis and food allergy,” says Boeckxstaens.
Source: Courtesy of Guy Boeckxstaens
Guy Boeckxstaens, a neurogastroenterology researcher at the Translational Research Center for GI Disorders (TARGID) at University Hospital Leuven in Belgium, says bioelectronic medicine is a more natural approach to treating disease, compared to using drugs that may act on many receptors and places
There are already upwards of 30 different visceral nerves that researchers are targeting with stimulation or blocking to treat various acute and chronic diseases
“The vagus nerve has been an obvious early target for bioelectronic medicines given it’s implicated in the autonomic regulation of virtually all visceral organs and is relatively easy to access. [But] it is certainly not the only nerve of interest,” says Eba. “By our count there are already upwards of 30 different visceral nerves that researchers are targeting with stimulation or blocking to treat various acute and chronic diseases.”
Silvia Conde and her colleagues at CEDOC, a chronic diseases research centre based in Lisbon, for example, have found that cutting the carotid sinus nerve prevents the development of insulin resistance, hypertension and obesity caused by high calorie diets[6]
. Now, with Galvani, they are studying the potential of treating type 2 diabetes by using a miniaturised wireless device to modulate the carotid sinus nerve. “We believe that bioelectronic therapies may offer a safe alternative to treat the pandemic of metabolic diseases,” says Conde.
Source: Courtesy of Silvia Conde
Silvia Conde at CEDOC, a chronic diseases research centre based in Lisbon, believes that bioelectronic therapies may offer a safe alternative to treat the pandemic of metabolic diseases
Time to refine
Despite promising advances, availability of bioelectronic medicines is not just around the corner. The Frost & Sullivan TechVision Healthcare team estimates that it will be five to seven years before the first bioelectronic medicines appear on the market and even longer before they become part of the medical mainstream.
Before they can become first-line and second-line therapies, “larger scale and longitudinal studies in specific populations are required to demonstrate long-term tolerability, safety and effectiveness, which will take time”, says Joshi.
“The question that should be asked is what do bioelectronic medicines need to do to be treated more like pharmaceuticals and less like existing neuromodulation devices (which are often the treatment of last resort) by patients, physicians and payers,” says Eba. “I believe the answer is that they will need to be miniaturised such that they are not daunting for the patient and easily implanted by surgeons or even the treating physician.”
Boeckxstaens says that although the potential application of the approach is huge, knowledge is still limited.
We know how to modify or stimulate nerves, but to improve the outcome of bioelectronic medicines we need more insight into pathway activation and which nerves to stimulate
“We know how to modify or stimulate nerves, but to improve the outcome of bioelectronic medicines we need more insight into pathway activation and which nerves to stimulate,” he says. “There is definitely interesting ongoing research and data collection but it is still a very grey area. There is a lot of work to be done.”
Nevertheless, Frost & Sullivan believes the convergence of electronics and sensors with pharmaceuticals is inevitable. There are already several pharmaceutical and MedTech companies that have invested in developing ingestible and implantable sensors for measuring physiological parameters. The next logical step would be the modulation and control of those physiological parameters, they say, adding that companies such as Johnson & Johnson and Boehringer Ingelheim have strategic investments in start-ups that are capable of developing miniature sensors and stimulators. “These are strong indications that pharmaceutical companies may indeed venture into bioelectronic medicines,” says a spokesperson for the Frost & Sullivan team. “GSK’s presence in this space is particularly interesting because they do not appear to be viewing bioelectronics as a threat, but rather as the future of pharmaceuticals.”
“It is very likely that more big pharma will diversify into bioelectronic medicine because of the considerable therapeutic potential with development in this area, both for the currently targeted space and for new areas of healthcare,” says Olofsson. With bioelectronic medicine, the convergence of molecular medicine, neuroscience, biology, electronics and computing, he foresees increasing numbers of joint ventures between the pharmaceutical industry, engineering and software companies, academia and the healthcare industry.
“If the field starts to grow into the new treatment paradigm that GSK and the NIH, among others, speak of, then it is inevitable that other big pharma will follow,” says Eba. “Look at what happened to the small molecule-focused pharmaceutical industry after Genentech led the way with the launch of large molecule biopharmaceuticals in the 1980s.”
Serdijn agrees: “I think pharma perceives electroceuticals as a game changer.”
References
[1] Andersson U & Tracey KJ. Neural reflexes in inflammation and immunity. J Exp Med 2012;209:1057–1068. doi: 10.1084/jem.20120571
[2] Huston JM, Ochani M, Rosas-Ballina M et al. Splenectomy inactivates the cholinergic antiinflammatory pathway during lethal endotoxemia and polymicrobial sepsis. J Exp Med 2006;203:1623–1628. doi: 10.1084/jem.20052362
[3] Meregnani J, Clarençon, Vivier M et al. Anti-inflammatory effect of vagus nerve stimulation in a rat model of inflammatory bowel disease. Auton Neurosci 2011;160:82–89. doi: 10.1016/j.autneu.2010.10.007
[4] Koopman FA, Chavan SS & Miljko S. Vagus nerve stimulation inhibits cytokine production and attenuates disease severity in rheumatoid arthritis. PNAS 2016;113:8284–8289. doi: 10.1073/pnas.1605635113
[5] Borovikova LV, Ivanova S, Zhang M et al. Vagus nerve stimulation attenuates the systemic inflammatory response to endotoxin. Nature 2000;405:458–462. doi: 10.1038/35013070
[6] Ribeiro MJ, Sacramento JF, Gonzalez C et al. Carotid body denervation prevents the development of insulin resistance and hypertension induced by hypercaloric diets. Diabetes 2013;62:2905–2916. doi: 10.2337/db12-1463