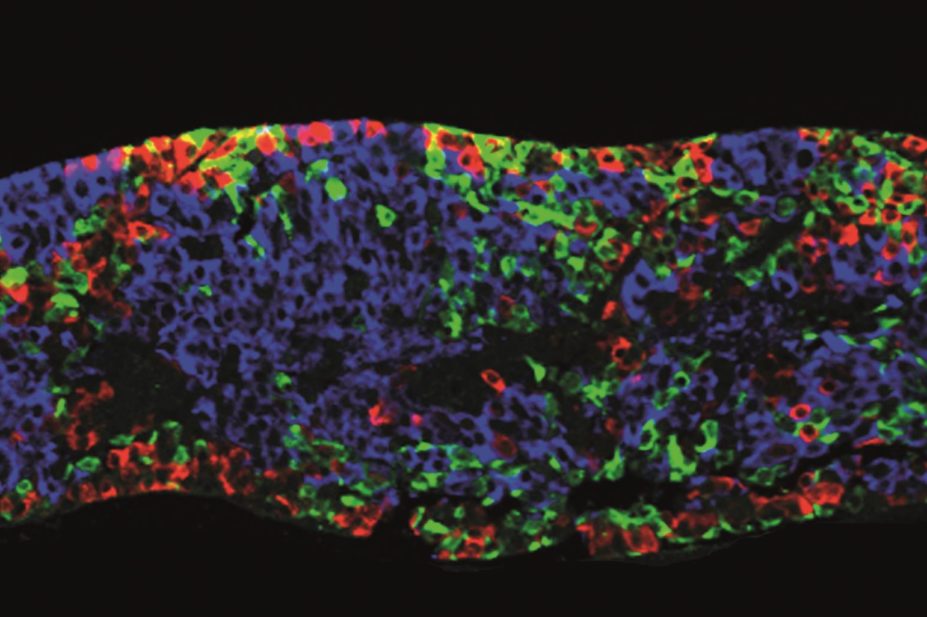
ViaCyte
People with type 1 diabetes lack the β cells in the pancreas that secrete insulin. So researchers have been trying to find a way of replacing these β cells and protecting them from attack by the immune system.
Patients with this autoimmune disease currently require daily insulin injections to regulate their glucose levels because their own insulin-producing cells have been destroyed. It is estimated that 387 million people worldwide are living with diabetes[1]
, with type 1 diabetes accounting for between 5% and 10% of cases[2]
.
For half a century, the best hope for treatment has been the transplantation of β cells from people who have recently died, and some patients have remained insulin-independent for as long as a year after the procedure[3]
[4]
. However, β cells from deceased donors are in short supply, and even when they are available, transplantation of these islet cells requires chronic immunosuppressive therapy to stop them being destroyed.
These types of transplants, involving β cells from organs of the deceased, gave us an important proof of concept
“These types of transplants, involving β cells from organs of the deceased, give us an important proof of concept that what we call ‘cell replacement therapy’ could be used to essentially replace missing endogenous β cells, if we had a renewable, unlimited source of β cells,” says Jeffrey R. Millman, a postdoctoral research fellow at Harvard Department of Stem Cell & Regenerative Biology (HSCRB) in Cambridge, Massachusetts.
In a bid to provide that supply, several research groups have been trying to create replacement β cells using human embryonic stem cells as a starting point. They have recently had some success, providing hope that one day millions of people with this condition may be able to produce insulin through cell transplantation without needing insulin injections.
Researchers from Harvard University reported in October 2014 that they had succeeded in creating, for the first time, viable embryonic stem-cell-derived β cells in vitro in the massive quantities required for cell transplantation[5]
.
“The entire process is synthetic and artificial, from the stem cells to the culture, to the differentiation among cells,” says Millman. “What’s biological here is the process that goes on in the cells, which have developmental programs inside them. And now, a laboratory-generated supply of β cells is on track to change the lives of patients with this condition.”
The Harvard research was spearheaded more than 20 years ago by Doug Melton, co-chair at the HSCRB, who was committed to finding a treatment because his then infant son had type 1 diabetes. Researchers published a study in 1998 heralding the production of embryonic stem cells in the laboratory[6]
, but no one knew how to unlock the potential of these pluripotent cells that could be programmed to morph into any cell of the body. “Back then, there was no published protocol on how to coax them, though differentiation, into a more restricted fate, such as that of a functional β cell,” says Millman.
For many years, numerous research groups have reported the ability to generate dysfunctional hormone-producing cells. “But those cells’ insulin output was not in response to high glucose levels — it was more like a firehouse sending out firemen whether or not there was a fire,” says Millman. After the Harvard researchers failed to turn these polyhormonal cells into mature β cells, they refocused their attention on pancreatic progenitor cells — stem cells that can become endocrine, exocrine or ductal cells. These progenitor cells could, when transplanted into mice, spontaneously turn into β cells, and produce insulin in the presence of glucose.
Millman and his colleagues spent years experimenting with a ‘combinatorial’ process, applying various chemicals to the progenitor cells over a calculated period of time to manipulate the developmental program within them. Then, two years ago, they noticed something they had not observed before in vitro. “We saw that some of our cells were expressing markers of β cells and seemed to be behaving like β cells,” says Millman. The researchers had succeeded in creating, for the first time, viable embryonic stem-cell-derived β cells in vitro.
To confirm their functionality, the β cells were first transplanted into non-diabetic mice, and were found to be glucose-responsive within two weeks of the transplant. After this success, the team transplanted the β cells into non-type 1 diabetic mice. The cells produced insulin within two weeks, and continued to do so for 16 weeks. In further studies, yet to be published, the transplanted β cells produced insulin for as long as six months.
Next on Harvard’s agenda is to improve β-cell differentiation procedures and conduct transplants on monkey models. Millman explains that, because type 1 diabetes is an autoimmune disease, any implanted stem-cell-derived β cells could be rejected. To overcome this, the team is collaborating with researchers at other institutions, including Massachusetts Institute of Technology, to develop ways to protect the β cells once they have been implanted.
“The approach that has the most traction is to encapsulate these β cells in an immune-system-shielding device,” Millman says. “Over the next several years, after we’ve successfully implanted encapsulated β cells in monkeys, we’ll hopefully proceed to human implants,” he says. “Within the decade, the hope is that such human under-the-skin implants — which could last several years, with periodic replacements — might take place in an out-patient setting.”
Maturing in vivo
Meanwhile, at stem-cell company ViaCyte, in San Diego, California, researchers are taking a different approach, implanting the pancreatic progenitor cells directly into patients. These cells then become insulin-producing islet cells in vivo.
ViaCyte is currently conducting a phase I/II clinical trial to evaluate its product, VC-01, in patients with type 1 diabetes.
“We stop at the precursor step, where the cell is already part of the pancreas, because of the shorter period of time — 12 days — to arrive there,” says Kevin D’Amour, ViaCyte’s chief scientific officer. “To take it to the β-cell level, you’d need nearly triple the time to allow the cells to further differentiate to produce insulin on their own.”
These implanted cultures differentiated, matured and effectively made functional human islet tissue in the mice
In 2008, ViaCyte published research explaining how it had taken embryonic stem cells to the progenitor level in vitro, and implanted those cultures into male mice[7]
. “These implanted cultures differentiated, matured and effectively made functional human islet tissue in the mice,” says D’Amour, who was a co-author on the study.
The cells were implanted at three different sites with different delivery systems: under the skin through needle injection; in the kidney capsule through a small tube that slowly infused the aggregates; and in the epididymal fat pads using structural scaffolding to hold the progenitor cells in place. “This study demonstrated that in 92% of all mice in the study, the implanted pancreatic progenitor cells became islets, which produced therapeutic levels of insulin,” says D’Amour.
Over the following six years, ViaCyte researchers continued to achieve reproducible results using mice, and scaled up their cell manufacturing process “to make billions, not just millions, of cells that could be tested on humans”, says D’Amour.
During this period, the team also focused on creating an encapsulation device for delivery. “We knew we’d have to protect cells from the immune system, and encapsulation was always the first choice,” he says. They opted for macroencapsulation, which can now hold 40 million cells, all of which can communicate with each other, but which are physically isolated from the patient’s immune system.
D’Amour admits that some researchers think that a cell-replacement product based on a pancreatic precursor, such as ViaCyte’s VC-01, risks being inconsistent. “Some would like to see the progenitor cells advanced to β cells to help ensure reliability,” he says. “But we don’t know if this is the case.” That’s why ViaCyte’s clinical trial, which began in September 2014, is so important. It is the first step towards receiving US Food and Drug Administration (FDA) approval for the product in the ‘biologic pharmaceutical’ category.
Enrolment for the trial is ongoing but is expected to total 40 adults with type 1 diabetes. Three patients have already been implanted with VC-01 at multiple sites under the skin. The patients, who continue to inject pharmaceutical insulin as needed, receive comprehensive medical supervision to measure insulin quantities resulting from the implant.
“That’s one of the reasons we’re moving so slowly,” says D’Amour. “We want to establish how this product is going to work in humans, and what the time course is for the cells inside the device to continue differentiation and ultimately produce therapeutic levels of insulin.” He expects that the subjects will end up using increasingly less pharmaceutical insulin. “We’ve designed this phase of the clinical trial so that the second cohort of participants will get a cellular dosage based on what we’ve learned about safe but therapeutic doses from the first cohort.”
The researchers are using C-peptide as a biomarker for endogenous insulin production because it is not produced as a result of injecting pharmaceutical insulin. “The C-peptide fragment has nothing to do with insulin functionality but, if you measure this substance,” says D’Amour, “then you know the body is endogenously producing insulin.”
ViaCyte has set 6 months after implantation as the interim time point for evaluating the efficacy of VC-01. “We don’t know yet how long the transplants will last in humans,” explains D’Amour. “What we did learn from our preclinical mouse studies is that the product functioned through the typical one-year lifespan of the mouse, which means it could have lasted even longer if the mice had longer life spans.”
Pending the results of this trial, the company hopes to have the green light from the FDA to proceed to a larger trial by the end of 2016.
Looking to the future, D’Amour talks about the potential distribution and widespread use of the product. “At ViaCyte we now have both frozen cells in liquid nitrogen and sterilised macroencapsulation devices,” he says. “We manufacture our final product over a three-day time frame — putting the cells in the device, sealing it, packaging it — so that it’s stable for seven days.”
D’Amour adds that ViaCyte is working on extending the product’s shelf life. He envisages that one day it will be available from hospitals, where new and replacement implants could be fitted in a non-invasive way. Implantation currently requires the expertise of a surgeon, but D’Amour hopes that in the future endocrinologists could be trained in the procedure.
Refining the protocol
Pancreatic progenitor cells are also the basis of research by a collaboration between BetaLogics, a division of pharmaceutical company Johnson & Johnson’s Janssen Research & Development in Raritan, New Jersey, and a group from the University of British Columbia (UBC) in Canada. In June 2012, the BetaLogics-UBC team published a study describing its four-stage protocol to produce pancreatic progenitors from human embryonic stem cells[4]
.
“Our findings, as reported in that study, are similar to those of ViaCyte’s and their approach,” says Timothy Kieffer, leader of UBC’s diabetes research group. “However, while ViaCyte transplanted the cells into healthy animals, we transplanted them into diabetic animals, and demonstrated that this approach effectively treated diabetes after an approximately four-month maturation period.”
The following year, the BetaLogics–UBC team published further research that used macroencapsulation devices as part of the transplantation into mice[5]
.
In September 2014, building on its four-stage protocol to make pancreatic progenitors, the team published further research describing a more advanced seven-stage protocol that converts human embryonic stem cells into insulin-producing cells, with stage-seven (S7) cells expressing key markers of mature pancreatic β cells[10]
.
These cells may gain these characteristics soon after transplant, and are able to reverse diabetes much faster than with cells obtained using our earlier progenitor protocol
The latest research involves the generation of more differentiated cells that more closely resemble mature β cells. They secrete insulin in response to glucose, which is similar to the results obtained by the Harvard researchers. “Upon closer examination, we determined that the cells were missing some characteristics of mature, human β cells,” says Kieffer. “However, these cells may gain these characteristics soon after transplant, and are able to reverse diabetes much faster than with cells obtained using our earlier progenitor protocol.”
The partnership is continuing to investigate encapsulation approaches, with macroencapsulation also serving as a container for the cells, which could be useful if the cells stop functioning optimally and require removal. “Future studies will assess the performance of our more mature S7 cells following encapsulation, and also evaluate whether encapsulation will protect the cells from immune attack,” says Kieffer. He points out that the date of a future clinical trial depends on the results of ongoing research, including safety and efficacy testing.
Screening for success
Millman explains that a further reason for developing the stem cells into β cells before implantation is so they can be used for drug screening. “Using these β cells to help screen the efficacy of newly developed type 2 diabetes drugs, which aim to help patients’ endogenous β cells produce more insulin, opens an untapped universe,” he says.
Researchers can apply the experimental drug to a Petri dish containing stem-cell-derived β cells and then observe whether the drug causes the β cell to secrete more insulin. “If such drugs demonstrate functionality in vitro through this type of drug screening, you would then further test them so they might become drug candidates to be used in humans,” explains Millman.
For at least the past five years, encapsulation has been the primary issue surrounding a type 1 diabetes cell-therapy cure
The potential advantages for type 2 diabetes patients are undeniable, but many medical researchers are focusing instead on all the recent research into type 1 diabetes, and what it means for the future.
“For at least the past five years, encapsulation has been the primary issue surrounding a type 1 diabetes cell-therapy cure, because of the need to prevent the destruction of implanted β or progenitor cells by the patient’s immune system,” says Karin Hehenberger, a doctor with expertise in type 1 diabetes and founder of LyfeBulb, an educational and social platform for people with chronic diseases, including diabetes. However, she says, most of the research conducted in this area had focused on finding a way to generate cells capable of producing endogenous insulin in the body.
“We now believe that we have solved the cell problem,” Hehenberger says, “so ViaCyte’s having discovered a shielding protocol represents a message to other research companies to intensify their efforts tied to encapsulation, either biologically or mechanically.”
“If ViaCyte is successful, it means that our model can work, and that’s good for everyone’s success,” says D’Amour. “When you’re talking about 1.5 million patients with type 1 diabetes in the United States, and as many as 8 million or more worldwide, there’s room for multiple players.”
References
[1] International Diabetes Federation. IDF diabetes atlas 2014.
[2] American Diabetes Association. Diagnosis and classification of diabetes mellitus. Diabetes Care 2009;32:s62-67.
[3] Shapiro AMJ, Lakey JRT, Ryan EA et al. Islet transplantation in seven patients with type 1 diabetes mellitus using a glucocorticoid-free immunosuppressive regimen. New England Journal of Medicine 2000; 343:230–238. doi: 10.1056/NEJM200007273430401.
[4] Shapiro AMJ, Ricordi C, Hering BJ et al. International trial of the Edmonton Protocol for islet transplantation. New England Journal of Medicine 2006; 355:1318–1330. doi: 10.1056 1056/NEJMoa061267.
[5] Pagliuca FW, Millman JR, Gürtler M et al. Generation of functional human pancreatic β cells in vitro. Cell 2014; 159:428–439. doi: 10,1016/j.cell.2014.09.040
[6] Thomson JA, Itskovitz-Eldor J, Shapiro SS, et al. Embryonic stem cell lines derived from human blastocysts. Science 1998; 282:1145–1147. doi: 10.1126/science.282.5391.1145.
[7] Kroon E, Martinson LA, Kadoya K et al. Pancreatic endoderm derived from human embryonic stem cells generates glucose-responsive insulin-secreting cells in vivo. Nature Biotechnology; 26:443–452; published online 20 February 2008. doi: 10.1038/nbt1393.
[8] Rezania A, Bruin JE, Riedel MJ et al. Maturation of human embryonic stem cell-derived pancreatic progenitors into functional islets capable of treating pre-existing diabetes in mice. Diabetes 2012; 61:2016–2029. doi: 10.2337/db11-1711.
[9] Bruin JE, Rezania A, Xu J et al. Maturation and function of human embryonic stem cell-derived pancreatic progenitors in macroencapsulation devices following transplant into mice. Diabetologia. 2013; 56:1987–1998. doi: 10.1007/s00125-013-2955-4.
[10] Rezania A, Bruin JE, Arora P et al. Reversal of diabetes with insulin-producing cells derived in vitro from human pluripotent stem cells. Nature Biotechnology 2014; 32:1121–1133. doi: 10.1038/nbt.3033.