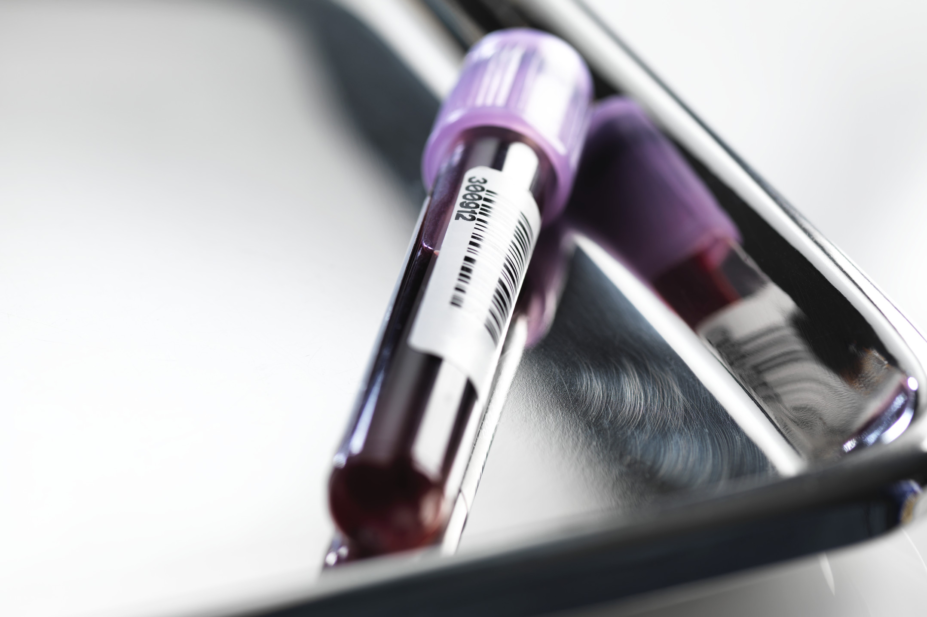
Science Photo Library
Anaemia (a low haemoglobin [Hb] level) is a complication of chronic kidney disease (CKD) that becomes more prevalent with advancing kidney dysfunction and is one of the most characteristic manifestations of CKD. People with kidney disease may develop anaemia associated with CKD as early as CKD Stage 3. However, CKD is more likely to be the cause of the anaemia at CKD Stage 4 onwards (or in CKD Stage 3b in people with diabetes), and accurate diagnosis of the cause of the anaemia is important to determine the correct treatment. Anaemia of CKD (ACKD) is essentially ubiquitous in those with end-stage kidney disease. More information on CKD stages, including their classification, is provided in Figure 1[1]
.
Figure 1: Prognosis of chronic kidney disease by glomerular filtration rate and albuminuria categories
Source: KDIGO 2012 Clinical Practice Guideline for the Evaluation and Management of Chronic Kidney Disease
GFR = glomerular filtration rate. Colour code: green = low risk (if no other markers of kidney disease, no chronic kidney disease); yellow = moderately increased risk; orange = high risk; red = very high risk. Reproduced with permission from KDIGO 2012 Clinical Practice Guideline for the Evaluation and Management of Chronic Kidney Disease[1]
The prevalence of ACKD is not well determined. The prevalence of CKD Stages 3–5 is estimated to be 6% of the UK population[2]
; people with this level of kidney impairment begin to develop anaemia associated with their CKD. Anaemia has a significant effect on quality of life for people with CKD, affecting exercise capacity, cognition and concentration. It can also have associated complications, including increased cardiac output, left ventricular hypertrophy and reduced immune responsiveness[3]
. Fortunately, treatment options, such as erythropoiesis-stimulating agents (ESAs) and iron therapies, are effective treatments for anaemia and offer symptomatic relief[4]
. This article provides an overview of the treatments for ACKD and the tests needed to prescribe them and assess their effectiveness.
Pathophysiology
In healthy individuals the kidneys play a vital role in maintaining Hb levels. The peritubular cells of the kidney produce the endogenous glycoprotein erythropoietin in response to the local hypoxia. Erythropoetin stimulates the bone marrow to produce red blood cells (erythrocytes) in a process known as erythropoiesis. Iron, a vital constituent of Hb — the oxygen-carrying protein found in erythrocytes — is incorporated into erythroid precursors in the bone marrow. Iron balance is maintained by the regulation of duodenal enterocytes (that absorb iron), macrophages (that recycle iron from senescent erythrocytes) and hepatocytes (that store iron). The majority of iron is found in Hb within circulating red blood cells, with the remainder stored as ferritin, primarily within the liver, or is bound to serum transferrin (the plasma transporter of iron).
CKD, and its associated inflammation, impairs the process of erythropoiesis and reduces iron availability to the bone marrow, which manifests as anaemia[3]
. In its simplest terms, ACKD can be described as a deficiency in erythropoietin or iron, but the pathophysiology is complicated by an inflammatory blockade; this is covered in ‘Hyporesponsiveness to anaemia treatment’.
Erythropoietin deficiency
The primary cause of anaemia in people with CKD is a deficiency in erythropoietin production, where the failing kidney produces insufficient erythropoietin to maintain adequate Hb levels. Treatment with an exogenous erythropoietin (an ESA) is usually effective at correcting the anaemia[5]
.
Iron deficiency
CKD is often associated with a negative iron balance. CKD affects iron absorption and is associated with increased blood-iron losses (including gastrointestinal), which deplete iron stores. Iron metabolism increases when there is an additional demand for iron in the bone marrow, which can occur when there is blood loss or when ESA therapy is given to drive erythropoiesis. If iron supply to the bone marrow cannot meet demand, either because of insufficient stores or mobilisation, then anaemia will develop[6]
. Iron-restricted erythropoiesis occurs either because iron stores to erythroid precursors in the bone marrow are exhausted or iron delivery cannot keep pace with the rate of erythropoiesis. Iron deficiency (ID) can be categorised as absolute or functional; often these coexist in CKD.
Absolute ID occurs when there are inadequate iron stores to meet the erythropoietic demand. A low serum ferritin level (<100µg/L) is a reliable indicator of absolute ID[7]
.
Functional ID occurs when there is an inadequate mobilisation of iron to the bone marrow despite apparently adequate body iron stores (as suggested by normal levels of serum ferritin). Iron delivery to erythroid precursors in the bone marrow cannot keep pace with the rate of erythropoiesis[7]
.
Diagnosis of ACKD
The 2012 Kidney Disease: Improving Global Outcomes clinical practice guideline for ACKD defines anaemia in adults as a Hb <130g/L in males and <120g/L in females[5]
. The 2017 UK Renal Association guidelines suggest that people with CKD should be investigated for the cause of their anaemia if their Hb is <110g/L or if they develop symptoms of anaemia[4]
. CKD should be considered as a possible cause of anaemia if glomerular filtration rate is less than 60ml/min/1.73m2 (CKD Stage 3a). However, CKD is more likely to be the cause of anaemia if renal function is:
- Less than 30ml/min/1.73m2 (CKD Stage 4); or
- Less than 45ml/min/1.73m2 (CKD Stage 3b) in people with diabetes;
and other potential causes of anaemia, for example, blood loss, vitamin B12 and folate deficiency, malnutrition and disorders of the bone marrow, have been excluded[4]
.
The assessment of ACKD should include:
- Hb concentrations — to assess to degree of anaemia;
- Red blood cell indices (mean cell volume and mean cell Hb) — to assess the type of anaemia;
- Absolute reticulocyte count — to assess erythropoietic activity;
- Serum ferritin — to assess iron stores;
- Tests to determine iron status;
- C-reactive protein — to assess inflammation[4],[8]
.
Iron-restricted erythropoiesis is common in ACKD, and can be observed clinically by the size (mean cell volume) and pallor of the red cells. Iron deficiencies produce a microcytosis (small cell volumes) as the erythrocyte’s precursors continue to divide to smaller cells in an attempt to concentrate the limited Hb. Often, deficiencies in folate or B12 coexist. Deficiencies in folate or B12 produce a macrocytosis (large cell volumes) as the erythrocyte’s precursors are deprived of key ingredients for cell division. Deficiencies in both iron and folate/B12 produce a mixed picture on the blood film; recognising and replacing the various deficiencies are essential elements of the treatment approach.
Determining iron status
In recent years, the role and management of ID in ACKD has become a prominent focus of clinical guidelines[3],[4]
. Assessing the person’s iron status is important for prescribing treatments and assessing their effectiveness. The standard tests used to diagnose ID, serum ferritin and transferrin saturation (TSAT) are surrogate biomarkers of iron storage and availability to the marrow; they do not directly measure iron incorporated in the erthyroid series and they are also affected by inflammation[3],[4]
. CKD is a pro-inflammatory state, which makes it difficult to diagnose ID using the standard tests. Iron levels in the blood drop during inflammation, mimicking some aspects of ID, as iron becomes ‘locked’ in the reticuloendothelial system. Ferritin is an acute phase reactant and is falsely elevated in the presence of inflammation or infection; however, it remains the most reliable marker of diagnosing absolute ID (when ferritin levels are <100µg/L) and can be used to monitor for potential iron overload (ferritin levels >800µg/L)[3],[4]
. C-reactive protein, as a marker for inflammation, is useful when interpreting the test for ID.
The National Institute for Health and Care Excellence (NICE) has updated its guidance for managing ACKD and now recommends that serum ferritin and TSAT are no longer used in isolation, but can be used in combination to assess ID[3]
. The guidelines suggest that either percentage hypochromic red blood cells (%HRC) or reticulocyte Hb content (CHr) are used first line[3]
. The tests provide a direct indication of the iron incorporation into erythrocyte precursors and therefore a better insight into possible iron-restricted erythropoiesis. These newer tests are not affected by inflammation in the same way as the standard tests. Both %HRC and CHr are limited by sample stability and test analyser availability, and neither are accurate in the presence of people with thalassaemia or thalassaemia trait[3]
. Figure 2 provides more information on the ID test measures on the erythropoietic timeline, while Table 1 provides a quick reference guide to the recommended tests for ID.
Figure 2: A visual representation of what the tests for iron deficiency measure along the erythropoietic timeline
Newer markers | Percentage of hypochromic red blood cells (%HRC) |
|
Reticulocyte haemoglobin (Hb) content (CHr) |
| |
Standard markers | Serum ferritin |
|
Percentage transferrin saturation (%TSAT) |
|
Managing ACKD
ESAs and iron therapy are well-established treatments for ACKD (see Figure 3). Before the introduction of these therapeutic tools, patients were treated with frequent blood transfusions. This was associated with complications, such as haemosiderosis, blood-borne infections and the development of anti-human leukocyte antibodies (HLA), which significantly reduced a patient’s chance of undergoing a successful renal transplant.
The important role of iron therapy in managing anaemia has been recognised over the past decade. Better diagnostic tests for ID and the response to iron therapy have helped shift attention towards iron treatment strategies. Intravenous (IV) iron therapy is the treatment of choice for people on haemodialysis, and oral iron may be effective in people who are not on haemodialysis; however, IV iron therapy is often required by patients receiving ESAs when oral iron has failed. Newer IV iron therapies that allow for higher doses of iron to be given over shorter periods of time and less frequently have helped improve the number of people that can be treated with IV iron, such is the demand on iron clinic capacity. Optimising the balance between iron repletion and erythropoiesis stimulation with ESAs has better outcomes for people with ACKD. When pharmacological therapies are effective, blood transfusions can be avoided and this is particularly important for people who are likely to receive a kidney transplant in the future.
Figure 3: Recent history of the treatments for chronic kidney disease
Iron therapy
Anaemia associated with CKD is often a function of ID and can therefore be corrected with iron therapy alone. Frequently, owing to the level of ID, IV iron is often required to meet the demand. When deciding on iron therapy, careful assessment of the potential benefits against the risks should be undertaken. IV iron may correct the symptoms of anaemia, reduce the need for ESA therapy and avoid the need for a blood transfusion. The risks of IV iron therapy, while rare, can be severe. There needs to be clear benefit for the patient when offering IV iron therapy and effective systems should be in place to manage any hypersensitivity reactions that may occur. The management approaches and considerations when using iron therapies are outlined below.
Oral iron
For people with CKD who are not on haemodialysis to attain and maintain Hb targets, oral iron should be considered. Oral iron at daily doses of 200–300mg elemental iron can be effective in treating ID and is a low-cost, safe and convenient option[9]
. IV iron may be required by people who do not tolerate oral iron, as well as by individuals who fail to attain Hb targets within three months of starting oral iron. However, once the iron deficit is corrected, oral iron may maintain Hb levels in the long term. For people treated with ESAs, the need for IV iron to attain or maintain Hb levels is more common. Oral iron is not always effective in treating ID, either because it is not tolerated, the absorption is hampered by inflammation of CKD, or the demand for iron is so great[3]
. However, oral iron should not be overlooked as a useful tool in the therapeutic approach in ACKD.
Intravenous iron
The approach to IV iron can be categorised as either:
- Low-dose high-frequency (LDHF) — 100–200mg and more than two infusions[3]
; - High-dose low-frequency (HDLF) — a minimum of 500mg per infusion and a maximum of two infusions[3]
.
The way that IV preparations available in the UK are categorised in the context of CKD is illustrated in Table 2.
LDHF generally have a lower purchase cost (per mg of elemental iron)[9]
and repeated infusions are required to address the iron deficit. The newer HDLF products generally have a higher purchase cost (per mg of elemental iron) but are more likely to correct iron deficits in a single infusion. However, if the iron deficit is greater than the maximum permissible dose of any one infusion, then repeated infusions may be necessary. The additional purchase costs of the HDLF preparations may be offset by the overall reduction in costs associated with less frequent per patient visits for IV iron and has the following advantages:
- The patient attends hospital less frequently for IV iron;
- Reduced frequency of venopuncture (an important consideration for those who may need a future arteriovenous fistula);
- Reduced demand on nursing and other healthcare resources (such as clinic capacity and transportation to and from the hospital).
For people on haemodialysis
IV iron is the treatment of choice for people on haemodialysis. ID develops owing to continuous blood losses, the widespread use of ESA therapy and the impaired absorption and release of iron from iron stores. This iron losses is typically between 2–3g per year and needs to be replaced with IV iron. Since there is ready IV access on haemodialysis, a LDHF IV iron regimen is recommended by the clinical guidelines. This typically consists of 100mg IV iron every two weeks. The exception to this is for home haemodialysis where the administration of IV iron is prohibited because of safety concerns[10]
. Home haemodialysis patients are required to attend clinics for IV iron and for logistical reasons are often treated with a HDLF approach.
For people not on haemodialysis
Provided it is effective and tolerable, oral iron is a convenient option for people who are not on haemodialysis. This includes people treated with ESA therapy who are likely to require long-term iron supplementation to maintain iron repletion. However, the demand for iron created by the ESAs increases the likelihood that IV iron is needed to replace or supplement the oral iron. When IV iron is required, clinical guidelines recommend a HDLF approach[3],[4]
.
Calculation of individual’s iron requirements is needed to guide the IV iron dose. One method of determining iron requirements is to use the Ganzoni formula[11]
:
Iron need = body weight [kg] x (target Hb [g/dl] – actual Hb [g/dl]) x 2.4 + iron for iron stores [mg iron]
In practice, the target Hb used in this equation is 100–120g/L for ESA-treated patients[3],[4],[12]
. Higher Hb targets of 120g/L or more are used for patients not on ESAs. The value used for the iron stores in the equation is 500mg for people over 35kg, but some clinicians may prefer to use a higher value that is determined by using 10–15mg/kg body weight. For obese patients, it is recommended that the ideal body weight is used.
Some renal units use a simplified approach to dosing HDLF iron by stratifying doses according to body weight and baseline Hb.
Intravenous iron | Formulation information | Product and manufacturer information | Comments |
---|---|---|---|
NICE considers HDLF to be a minimum of 500mg of iron in each infusion. This table considered the IV formulations in this context of the NICE definition. Refer to summary of product characteristics (SmPC) for full prescribing and administration information, as well as other methods of administration. *Refer to SmPC for the distinction between the administration during haemodialysis sessions and the administration (not while on haemodialysis) to a person receiving haemodialysis. †Products suitable for HDLF regimens as defined by NICE can also be used for LDHF regimens – see the SmPC. | |||
Low-dose high-frequency (LDHF) | Venofer®; Vifor Pharma UK Ltd (iron hydroxide sucrose complex) |
| |
Diafer®; Pharmacosmos UK Ltd (iron isomaltoside 1000) |
| ||
High-dose low-frequency (HDLF)â€
| Slower infusion formulation | Cosmofer®; Pharmacosmos UK Ltd (iron hydroxide dextran complex) |
|
Quicker infusion formulation | Monofer®; Pharmacosmos UK Ltd (iron isomaltoside 1000) |
| |
Quicker infusion formulation | Ferinject®; Vifor Pharma UK Ltd (ferric carboxymaltose) |
|
Risks of intravenous iron
The risks associated with IV iron may include both short-term immediate infusion reactions and longer term toxicities associated with iron; however, newer preparations are associated with lower frequencies of severe hypersensitivity reactions. Nevertheless, regulatory authorities stipulate that IV iron should only be administered in an environment where resuscitation facilities are available so that patients who develop an allergic reaction can be treated immediately[10]
. Healthcare professionals involved in the management of IV iron will be familiar with a European Medicines Agency assessment report for iron-containing IV medicinal products and the new strengthened recommendations to manage and minimise risk[10]
.
Hypersensitivity reactions
It is important to differentiate between severe hypersensitivity reactions, which are life-threatening and will contraindicate further IV iron administration, and minor infusion reactions (MIRs), which are not serious and can be managed by adjusting the IV iron prescription. MIRs are infrequent reactions attributable to the administration of IV iron. Symptoms may include mild urticaria, facial flushing, back or loin pain, or a mild arthralgia or myalgia of the chest[18],[19]
. These reactions are a likely consequence of free plasma iron release[19]
. MIRs can be managed by stopping the infusion for at least 15 minutes and the infusion restarted at 50% of the initial infusion rate[19]
. If the patient experiences any symptoms that suggest an allergic reaction, the infusion should be immediately stopped. Careful assessment of the patient’s symptoms should determine the reaction type and whether the infusion should recommence. All future IV iron, regardless of formulation, are contraindicated in people who experience severe hypersensitivity reactions to any IV iron.
Other risks
The long-term safety concerns of IV iron remain a subject of investigation. The use of IV iron is cautioned in acute or chronic infection; how to manage ID during periods of active infection is open to debate. It is common practice to withhold IV iron during active infection since iron is a substrate for bacterial growth and may interfere with the immune response by reducing the functionality of circulating granulocytes[20]
. Other concerns relate to the oxidative stress from labile iron that may result if ferritin and transferrin binding is oversaturated. These concerns are the subject of current research.
Erythropoiesis-stimulating agents
The primary cause of ACKD is a deficiency in erythropoietin[21]
. NICE recommends offering ESAs to people with ACKD, irrespective of CKD stage, or who are likely to benefit in terms of physical function and quality of life[3]
. In practice, this is typically when people with CKD reach Stage 3b. ESAs can improve quality of life, reduce hospital admissions and lower the requirement for blood transfusions[22]
. As previously discussed, optimising a person’s iron status improves the response to ESAs and thereby potentially reduces the associated CV risks[23]
. ESA therapy is usually provided by anaemia specialists within nephrology services, which are set up in a way that provides coordinated care in accordance with NICE guidelines, offering people flexible access to specialists to help manage their condition. Before initiating ESAs, clinicians should evaluate iron status, correct any deficiency and maintain iron repletion throughout ESA therapy. The coordinated care model also provides IV iron services as part of the anaemia treatment package.
ESAs can be broadly categorised into short- and longer-acting agents. All ESAs are equally effective.
Short-acting ESAs
The first-generation ESAs, epoetin alfa (Eprex®; Janssen-Cilag Ltd) and beta (NeoRecormon®; Roche Products Ltd), have shorter half-lives and require more frequent dosing. This is typically 2–3 times per week in the correction phase and may be extended to once per week, or even once per fortnight, in the maintenance phase; extending to this interval usually requires a higher overall dose. When epoetins are given subcutaneously, a lower dose is required compared with being given by the IV route[24],[25]
. Epoetins have been available for nearly 30 years and are now available as biosimilars. Pharmacists are reminded that the prescribing of biosimilars should be by brand or with the manufacturer as a suffix[26]
.
Longer-acting ESAs
The second-generation ESA darbopoetin alfa (Aranesp®; Amgen Ltd) and the third-generation ESA methoxy polyethylene glycol-epoetin beta (Mircera®; Roche Products Ltd) are longer acting and require less frequent administration, extending to fortnightly or monthly dosing. Unlike the shorter-acting epoetins, the route of administration — subcutaneous or IV — does not affect the dose requirement. As a rule, the dose conversion between the short-acting and longer-acting ESAs is 200 IU:1µg, although this is influenced by route and frequency of administration.
Choice of ESA
Since all ESAs are equally effective at maintaining Hb levels, the choice of ESA will be influenced by the dialysis status, the local availability of ESAs and the cost, the preferences of the individual, and whether they are able to self-administer. For people on haemodialysis, it may be better to give ESAs through the IV route via the dialysis machine and, owing to the regularity of dialysis, the frequent administration of a short-acting ESA would not be an inconvenience. For those not on haemodialysis, ESAs are administered subcutaneously. These people may prefer less frequent injections and may benefit from a longer-acting ESA. A long-acting ESA is the treatment of choice when the person cannot self-administer and is dependent on a healthcare professional to do so.
Optimal Hb with ESAs
The correction to normal levels of Hb with ESAs is not usually recommended in people with ACKD. Typically, aspirational Hb is maintained between 100–120g/L for adults, as defined in both clinical and regulatory guidelines[3],[4],[12]
. Lower levels may be agreed if high doses of ESAs are required to maintain the aspirational range. The Hb target range applies exclusively to patients receiving ESAs; it is not intended to apply to the treatment of ID in people receiving iron therapy without the use of ESAs.
Maintaining adequate Hb relieves people with ACKD of their symptoms. However, close monitoring of patients receiving ESAs is required to ensure Hb levels do not rise too rapidly (a rise of >10–20g/L/month) or significantly above the upper Hb threshold, especially if high ESA doses are required[3],[4]
. Evidence from three large randomised controlled trials, CHOIR, CREATE and TREAT, suggested there is no benefit in titrating to higher Hb targets and doing so increases the risk of harm from ESA therapy[27],[28],[29]
(see Figure 3).
The doses used to attain or maintain Hb level is directed by local protocol but will consider current Hb level, target Hb level, the observed rate of increase in Hb, and clinical circumstances. By contrast, the manufacturers’ summary of product characteristics suggest initial dosing according to the person’s weight[30],[31],[32]
. Hb should be monitored every two to four weeks in correction phases and every one to three months for stable patients in the maintenance phase[4]
with regular blood pressure monitoring. ESA doses may be adjusted in response to Hb level either by changing the syringe strength (typically by 20–30%) or by adjusting the interval between doses.
Hyporesponsiveness to anaemia treatment
As described, the pathophysiology of ACKD is more complicated than simple erythropoietin or ID. In CKD there is often a complex inflammatory state that interferes with normal erythropoiesis, despite treatment with ESA and iron. Some people fail to respond to even very large doses of ESAs, while others may fail to respond to oral iron supplementation. Hyporesponsiveness to treatment may be acute or chronic in nature. This inflammatory state also interferes with the tests for iron deficiencies, which makes the treatment decision more difficult.
Disease states involving infection or inflammation increase expression of hepcidin, a protein synthesised in the liver that regulates plasma iron levels and iron metabolism[33]
. Hepcidin inhibits the mobilisation of iron from stores such as hepatocytes, enterocytes and macrophages, and reduces absorption of iron from the gastrointestinal tract[34]
.
Proinflammatory cytokines, namely IL-6, disrupt iron metabolism and antagonise the effects of erythropoietin by directly inhibiting erythoid progenitor (an erythrocyte prescursor) cell growth[35],[36]
.
Acute hyporesponsiveness is often seen during periods of intercurrent illness, such as infections or relapses in inflammatory diseases. Increasing the ESA dose in these instances is often futile and may even be a risky treatment approach[3]
. Infections and active inflammation usually means IV iron therapy is avoided, either because iron has the potential to interfere with the immune response or because a raised ferritin level prohibits IV iron administration. Not giving the IV iron hampers effective erythropoiesis. Often the anaemia remains uncorrected until the acute causes for hyporesponsiveness have resolved. If the anaemia is severe, treatment with blood transfusions may be necessary. Healthcare professionals should also be mindful of the rare but serious possibility of ESA-induced pure red cell aplasia, which is characterised by a sudden drop in Hb without a drop in platelets and white cell counts[4]
.
Chronic hyporesponsiveness or even frank ESA resistance has different causes to acute hyporesponsiveness. Inflammation of chronic disease, such as CKD, can reduce production of and impair the effects of erythropoietin on the bone marrow. Increased hepcidin production reduces iron absorption and mobilisation, further impairing erythropoiesis. Chronic inflammatory disorders also reduce mean red cell survival[37]
.
The Renal Association defines ESA hyporesponsiveness (‘resistance’) as failure to reach Hb target despite a subcutaneous epoetin dose exceeding 300IU/kg/week[4]
. These patients should be investigated for hyporesponsiveness, which can often be corrected by giving iron therapy, correcting B12 or folate deficiencies, treating hidden infections, or for those on dialysis by optimising dialysis adequacy. Patients should be referred to a haematology service when the cause of ESA resistance cannot be identified or an underlying haematological disorder is suspected[3]
.
- This article was amended on 17 September 2018 to amend the definition of hyperchromic red cells in column 2 of Table 1 and amend the title of Figure 3.
- This article was amended on 2 April 2019 to correct hyperchromic to hypochromic in Table 1
Emerging and future therapies
Other treatments for anaemia of chronic kidney disease are currently being explored.
Ferric pyrophosphate citrate
Intended for treating iron deficiency in people receiving haemodialysis, ferric pyrophosphate citrate is a water soluble, carbohydrate-free, iron salt that is added to the dialysis fluid[38]
.
Hypoxia-inducible factor stabilisers
Hypoxia-inducible factors (HIFs) induce the transcription of certain genes in response to hypoxia, resulting in increased synthesis of erythropoietin and enhanced iron mobilization[39]
. HIF stabilisers prevent the degradation of HIF[39]
.
Hepcidin antagonists
Hepcidin produced by the liver regulates iron availability. High hepcidin, as seen in inflammation, reduces iron absorption and release from stores. Hepcidin antagonists inhibit the action of hepcidin.
Financial and conflicts of interest disclosure:
The authors have no relevant affiliations or financial involvement with any organisation or entity with a financial interest in or financial conflict with the subject matter or materials discussed in the manuscript. No writing assistance was used in the production of this manuscript.
Reading this article counts towards your CPD
You can use the following forms to record your learning and action points from this article from Pharmaceutical Journal Publications.
Your CPD module results are stored against your account here at The Pharmaceutical Journal. You must be registered and logged into the site to do this. To review your module results, go to the ‘My Account’ tab and then ‘My CPD’.
Any training, learning or development activities that you undertake for CPD can also be recorded as evidence as part of your RPS Faculty practice-based portfolio when preparing for Faculty membership. To start your RPS Faculty journey today, access the portfolio and tools at www.rpharms.com/Faculty
If your learning was planned in advance, please click:
If your learning was spontaneous, please click:
References
[1] KDIGO 2012 Clinical Practice Guideline for the Evaluation and management of chronic kidney disease. Chapter 1: Definition and classification of CKD. Kidney Int Suppl 2013;3(1):19–62. Available at: https://www.sciencedirect.com/science/article/pii/S2157171615311011#f0045 (accessed September 2018)
[2] Public Health England. 2014. Chronic Kidney Disease Prevalence Model. Available at: https://www.gov.uk/government/uploads/system/uploads/attachment_data/file/612303/ChronickidneydiseaseCKDprevalencemodelbriefing.pdf (accessed September 2018)
[3] National Institute for Health and Care Excellence. Guideline on anaemia management in chronic kidney disease. 2015. Available at: http://www.nice.org.uk/guidance/NG8/evidence (accessed September 2018)
[4] Mikhail A, Brown C, Williams J et al. Clinical Practice Guideline: Anaemia of Chronic Kidney Disease. Renal Association. 2017. Available at: https://renal.org/wp-content/uploads/2017/06/anaemia-of-chronic-kidney-disease5d84a231181561659443ff000014d4d8.pdf (accessed September 2018)
[5] KDIGO Clinical Practice Guidelines for Anaemia in Chronic Kidney Disease. Kidney Int Suppl 2012;2(4). Available at: http://www.kdigo.org/clinical_practice_guidelines/pdf/KDIGO-Anemia%20GL.pdf (accessed September 2018)
[6] Macdougall IC, Hutton RD, Cavill I et al. Poor response to treatment of renal anaemia with erythropoietin corrected by iron given intravenously. BMJ 1989;299(6692):157–158. PMC1837074
[7] Urrechaga E, Borque L & Escanero J. Biomarkers of hypochromia: the contemporary assessment of iron status and erythropoiesis. Bio Med Res Int 2013;2013:603786. doi: 10.1155/2013/603786
[8] Buttarello M, Pajola R, Novello E et al. Diagnosis of iron deficiency in patients undergoing hemodialysis. Am J Clin Pathol 2010;133(6):949–954. doi: 10.1309/AJCPQAX0JFHFS0OA
[9] Joint Formulary Committee. 2017. British National Formulary. [ed. 74] ed. London: BMJ Group and Pharmaceutical Press
[10] European Medicines Agency. Assessment report for: Iron containing intravenous (IV) medicinal 12 products, 2013. Available at: http://www.ema.europa.eu/docs/en_GB/document_library/Referrals_document/IV_iron_31/WC500150771.pdf (accessed September 2018)
[11] Baird-Gunning J & Bromley J. Correcting iron deficiency. Aust Prescr 2016;39(6):193–199. doi: 10.18773/austprescr.2016.069
[12] Medicines and Healthcare products Regulatory Agency. Epoetins for the management of anaemia in patients with chronic renal disease: mortality and cardiovascular morbidity. MHRA Public Assessment Report 2017. Available at: http://www.mhra.gov.uk/home/groups/s-par/documents/websiteresources/con2033215.pdf (accessed September 2018)
[13] Summary of Product Characteristics. 2016. Venofer: iron sucrose. Vifor Pharma UK Ltd
[14] Summary of Product Characteristics. 2014. Diafer: iron (III) isomaltoside 1000. Pharmacosmos UK Ltd
[15] Summary of Product Characteristics. 2014. Cosmofer: iron dextran. Pharmacosmos UK Ltd
[16] Summary of Product Characteristics. 2014. Monofer: iron (III) isomaltoside 1000. Pharmacosmos UK Ltd
[17] Summary of Product Characteristics. 2017. Ferinject: Vifor Pharma UK Ltd
[18] Rampton D, Folkersen J, Fishbane S et al. Hypersensitivity reactions to intravenous iron- guidance for risk minimization and management. Haematologica 2014;99(11):1671–1676. doi: 10.3324/hadatol.2014.111492
[19] Auerbach M & Macdougall I. Safety of intravenous iron formulations: facts and folklore. Blood Transfus 2014;12(3):296–300. doi: 10.2450-2014
[20] Brookhart M, Freburger J, Ellis A et al. Infection risk with bolus versus maintenance iron supplementation in hemodialysis patients. J Am Soc Nephrol 2013;24:1151–1158. doi: 10.1681/ASN.2012121164
[21] National Kidney Foundation Kidney Disease Outcome Quality Initiative Advisory Board. K/DOQI clinical practice guidelines for chronic kidney disease: evaluation, classification, and stratification. Kidney Disease Outcome Quality Initiative. Am J Kidney Dis 2002;39(Suppl 2):S1–246. Available at: http://www.kidney.org/professionals/kdoqi/guidelines_ckd/toc.htm (accessed September 2018)
[22] Jones M, Ibels L, Schenkel B & Zagari M. Impact of epoetin alfa on clinical end points in patients with chronic renal failure: a meta-analysis. Kidney Int 2004;65(3):757–767. doi: 10.1111/j.1523-1755.2004.00450.x
[23] Agarwal R. Management of anaemia in CKD the relative importance of erythropoeitin and iron. Nephol Dial Trans 2011;4(1):i1–i2. doi: 10.1093/ndtplus/sfr043
[24] Tonelli M, Blake PG & Muirhead N. Predictors of erythropoietin responsiveness in chronic hemodialysis patients. ASAIO J 2001;47:82–85. PMID: 11199321
[25] Coladonato JA, Frankenfield DL, Reddan DN et al. Trends in anemia management among US hemodialysis patients. J Am Soc Nephrol 2002;13(5):1288–1295. PMID: 11961017
[26] Mikhail A & Brown C. Clinical efficacy and safety of biosimilar epoetin: focus on epoetin zeta. Dove Medical Press Limited. Volume 2015:5:65–73. doi: 10.2147/BS.S50456
[27] Singh AK, Szczech L, Tang KL et al., for the CHOIR Investigators. Correction of anemia with epoetin alfa in chronic kidney disease. N Engl J Med 2006;355(20):2085–2098. doi: 10.1056/NEJMoa065485
[28] Drüeke TB, Locatelli F, Clyne N et al., for the CREATE Investigators. Normalization of hemoglobin level in patients with chronic kidney disease and anemia. N Engl J Med 2006;355(20):2071–2084. doi: 10.1056/NEJMoa062276
[29] Goldsmith D & Covic A. Time to Reconsider Evidence for Anaemia Treatment (TREAT) = Essential Safety Arguments (ESA). Nephrol Dial Transplant 2010;25(6):1734–1737. doi: 10.1093/ndt/gfq099
[30] Summary of Product Characteristics. 2018. Mircera: methoxy polyethylene glycol-epoetin beta
[31] Summary of Product Characteristics. 2017. Eprex: epoetin alfa. Janssen-Cilag Ltd
[32] Summary of Product Characteristics. 2017. Aranesp: darbepoetin alfa. Amgen
[33] Stolan I, Manolescu B, Atanasiu V et al. Il-6-STAT-3-Hepcidin: linking inflammation to the iron metabolism. Rom J Intern Med 2007;45(3);305–309. PMID: 18333366
[34] Pietrangelo A. Hepcidin in human iron disorders: therapeutic implications. J Hepatol 2011;54(1):173–181. doi: 10.1016/j.jhep.2010.08.004
[35] Rossert J, Gassmann-Mayer C, Frei D & McClellan W. Prevalence and predictors of epoetin Hyporesponsiveness in chronic kidney disease patients. Nephrol Dial Transplant 2007;22:794–800. doi: 10.1093/ndt/gfl716
[36] Kalantar-Zadeh K, McAllister CJ, Lehn RS et al. Effect of malnutrition-inflammation complex syndrome on EPO hyporesponsiveness in maintenance hemodialysis patients. Am J Kidney Dis 2003;42(4):761–773. PMID: 14520627
[37] Adamson JW. Hyporesponsiveness to erythropoiesis stimulating agents in chronic kidney disease: the many faces of inflammation. Adv Chronic Kidney Dis 2009;16(2):76–82. doi: 10.1053/j.ackd.2008.12.009
[38] Shah HH, Hazzan AD & Fishbane S. Ferric pyrophosphate citrate: a novel iron replacement agent in patients undergoing hemodialysis. Semin Nephrol 2016;36(2):124–129. doi: 10.1016/j.semnephrol.2016.02.007
[39] Gupta N & Wish J. Hypoxia-inducible factor inhibitors: a potential new treatment for anemia in patients with CKD. Am J Kidney Dis 2017;69(6):815–826. doi: 10.1053/j.ajkd.2016.12.011