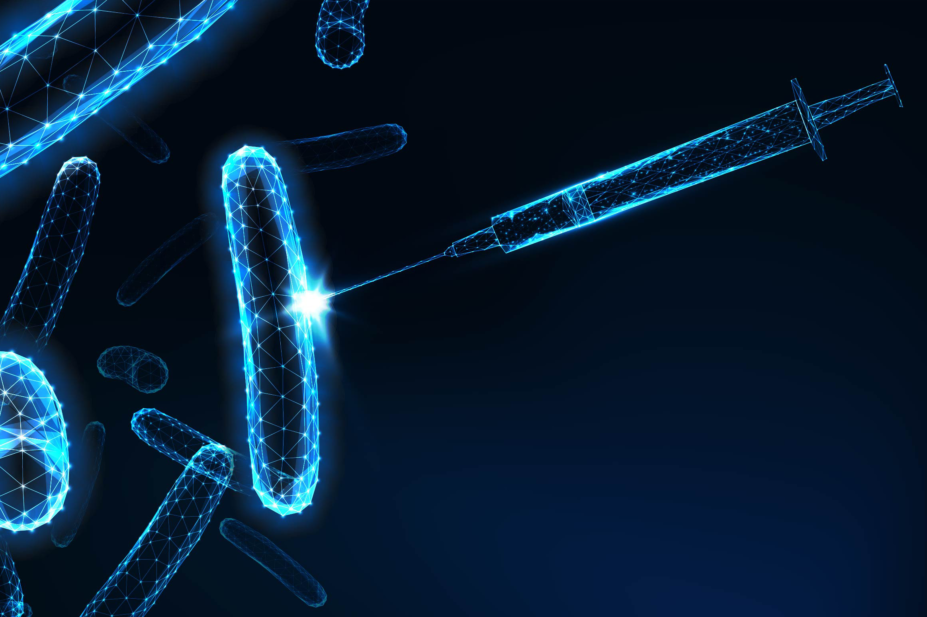
Mclean / Shutterstock.com
There is no doubt that antimicrobial resistance (AMR) is a problem, but there is concern over what policy makers and clinicians are doing about it. Governments and pharmaceutical companies could spend more public and private funds searching for new medicines, but history says that simply releasing new products will not be enough to tackle this problem — AMR develops too quickly. The time between first prescription of a medicine and first observation of resistance can be just a few years[1]
,[2]
. For some infections, such as gonorrhoea, drug after drug has been rolled out until untreatable disease has emerged[3]
.
Instead, efforts to tackle AMR would have more of an impact if closer attention was paid to the evolutionary biology that governs the emergence of resistance in bacteria. Here are five rules to guide future AMR strategies and help pharmacists to think about safe ways of diversifying antibiotic use[4]
.
Rule 1: prevention is better than a cure
If researchers were trying to start a resistance catastrophe, they would deploy a single antibiotic at a lethal dose to as many microbes as possible in the global population. In this situation, bacteria with genes that confer antibiotic resistance would thrive and rapidly dominate the population. This is the worst-case scenario, in which many microbes are exposed to high doses of the medicine, selection pressure is very high and —because all the microbes are exposed to a single drug class — the selection pressure is homogenous.
The most obvious improvement on this scenario is to reduce selection pressure by exposing as few microbes to the antibiotic as possible.
High levels of resistance are synonymous with multidrug resistance and can undermine mixing strategies
This becomes more complicated when there are multiple possible antibiotics that could be used to treat a given infection. One possible solution is using a single antibiotic at any given time and replacing it as soon as resistance renders it ineffective. Alternatively, for some infections, combinations of antibiotics could be used to reduce the probability of resistance evolving. Another approach is to increase the heterogeneity (diversity) of selection by prescribing different antibiotics to different parts of the population (known as ‘mixing’), or by using preferred antibiotics for short periods of time (known as ‘cycling’).
However, all three strategies are most beneficial when there are low frequencies of resistance[5]
. High levels of resistance are synonymous with multidrug resistance and can undermine mixing strategies. The heterogeneous patterns of antibiotic prescribing in mixing limit the transmission of resistant bacteria, but a person surrounded by others with a high resistance burden may readily acquire microbes that are resistant to many drugs.Fachi
Driving up resistance while using monotherapies before trying combinations is also a known risk factor for the emergence of multi-drug resistant infections, such as tuberculosis[6]
. In order to preserve the efficacy of new antibiotics, it is far better to act early than waiting for resistance to become a problem.
Rule 2: do not rely on a ‘fitness cost’
Microbes that have acquired resistance to antibiotics may have effectively paid a physiological price to do so. This trade-off is known as the ‘fitness cost’ — the ‘Achilles’ heel’ of bacteria.
Where antibiotics are not used, resistant bacteria can be outcompeted by bacteria that would be susceptible to the antibiotic, so fitness costs can be important for cycling strategies. But deploying strategies that depend purely on fitness costs can be unreliable. For example, fitness costs can be low, especially for bacteria with resistance genes on plasmids (small strands of DNA that replicate independently of chromosomes and can be transferred to other microbes)[7]
.
Long-term selection (over weeks or months, rather than years) can further reduce these costs, which gives another reason to act early. While the correlation between antibiotic use and prevalence of resistance is strong, withdrawing antibiotics does not always lead to falling resistance levels — potentially because many microbes are resistant to multiple drugs[8],[9]
.
Rule 3: control mutation supply
The development of resistance depends on a supply of genetic novelty. This can partly be controlled by people, for example, acting to limit the supply of mutations from agriculture.
Although the effects of antibiotic use in agriculture on resistance in humans are debated. A recent study of Escherichia coli in the UK argues that the resistance genes and bacterial genotypes in agriculture are distinct from those in human populations, and concludes that antibiotic use in agriculture is unimportant for antimicrobial stewardship[10]
. However, it is important to remember that agricultural use of the antibiotic colistin was a major factor in the transfer of resistance to humans[11]
,[12]
.
For many Gram-negative microbes, genetic novelty arrives neatly packaged on bacterial plasmids, and initial acquisition of new resistance genes in human-associated microbes is a very different process from their subsequent selection and increase in frequency.
The acquisition of new genes is dependent on the prevalence of resistance plasmids (or other mobile DNA) in the environment, in which agriculture plays a major role. Once these genes arrive in human-associated microbes, prescribing practice drives selection. Nevertheless, agricultural use can accelerate the initial appearance of resistance, and the example of colistin shows that it is unwise to indulge in large-scale use of novel drugs outside of human populations[12]
.
Rule 4: prescribe short courses
An antibiotic creates a selection pressure by killing non-resistant bacteria; this provides an opportunity for bacteria that are resistant to the antibiotic to thrive. This selection pressure can be reduced by not only reducing the number of prescriptions, but also by lowering the dose of antibiotics and/or reducing the duration of treatment.
The balance of evidence favours shorter courses rather than lower doses[4]
,[13]
,[14]
. Low doses can accelerate the appearance of resistance because there are simply many more mutations that can confer resistance to low doses. Low doses can also select for plasmid-borne resistance and fail to cope with pharmacogenetic variation[4]
,[15]
.
Rule 5: monitor local resistance levels
The worst-case scenario outlined above illustrates how homogeneity of prescribing practice is a bad idea. The situation is sometimes exacerbated by strong top-down guidelines on preferred treatments, but there are currently too few trials to help shape mixing or cycling strategies to increase heterogeneity. The latest theory suggests the best approaches are responsive, so information is vital in this effort; local levels of resistance should shape future drug preference[16]
.
Too many trials of resistance management interventions have paid little attention to fundamental principles
Too many trials of resistance management interventions have paid little attention to fundamental principles[4]
. For example, mixing and cycling should rotate structurally distinct drugs that can be overcome only by distinct resistance mechanisms[17]
. Cycling different beta-lactams that can all be overcome by AmpC beta-lactamases, for example, is unlikely to provide effective antimicrobial stewardship[4]
. While it may not be possible to predict whether cycling strategies will work in advance, it is a relatively low-risk intervention and a good monitoring strategy will soon provide data on whether this approach has been effective[16]
.
What clinicians and pharmacists can do
An important challenge for pharmacists is to think about safe ways of diversifying drug use. Heterogeneous prescribing can help to moderate selection for resistant bacteria, but practice must also respect the principles summarised above.
Recent research commissioned by Public Health England suggests many antibiotic courses are too long[13]
. The data justifying the length of antibiotic courses require careful examination[14]
.
Healthcare professionals also need to think beyond the hospital and life-threatening infections to the use of antibiotics in the community — which makes up 70% of all prescribed antibiotics — and pay close attention to the large number of self-limiting infections for which most patients do not require antibiotics[18]
.
Solutions to AMR must consider a broad range of interventions that focus efforts around problematic groups, in detailed management plans — i.e. they must be broad in approach but narrow in focus. This has proved effective in insecticide resistance management and in reducing the incidence of methicillin-resistant Staphylococcus aureus (MRSA)[13]
. Currently, the biggest challenge is presented by enteric Gram-negative Enterobacteriaceae, and this will require bold action.
Ben Raymond is associate professor of microbiology and entomology, University of Exeter; member, Cornwall Antimicrobial Resistance Group
Neil Powell is a consultant antimicrobial pharmacist, Royal Cornwall Hospitals NHS Trust; member, Cornwall Antimicrobial Resistance Group
Correspondence to: b.raymond@exeter.ac.uk
References
[1] Sun Y, Cai Y, Liu X et al. Int J Antimicrob Agents 2013;41(2):110–116. doi: 10.1016/j.ijantimicag.2012.09.005
[2] Clatworthy AE, Pierson E & Hung DT. Nat Chem Biol 2007;3(9):541–548. doi: 10.1038/nchembio.2007.24
[3] Bignell C & Unemo M. Int J STD AIDS 2013;24(2):85–92. doi: 10.1177/0956462412472837
[4] Raymond B. Evol Appl 2019;12(6):1079-1091. doi: 10.1111/eva.12808
[5] Bonhoeffer S, Lipsitch M & Levin BR. PNAS 1997;94(22):12106–12111. doi: 10.1073/pnas.94.22.12106
[6] Monedero I & Caminero JA. Ther Adv Resp Dis 2010;4(2):117–127. doi: 10.1177/1753465810365884
[7] Vogwill T & Maclean RC. Evol Appl 2015;8(3):284–295. doi: 10.1111/eva.12202
[8] Goossens H, Ferech M, Vander Stichele R et al. Lancet 2005;365(9459):579–587. doi: 10.1016/S0140-6736(05)17907-0
[9] Lipsitch M. Trends Microbiol 2001;9(9):438–44. doi: 10.1016/S0966-842X(01)02130-8
[10] Day MJ, Hopkins KL, Wareham DW et al. Lancet Infect Dis 2019; In press. doi: 10.1016/S1473-3099(19)30273-7
[11] Shen Z, Wang Y, Shen Y et al. Lancet Infect Dis 2016;16(3):293. doi: 10.1016/S1473-3099(16)00061-X
[12] Smith DL, Harris AD, Johnson JA et al. PNAS 2002;99(9):6434–6439. doi: 10.1073/pnas.082188899
[13] Spellberg B & Rice LB. Ann Int Med 2019;171(1):210–211. doi: 10.7326/M19-1509
[14] Pouwels KB, Hopkins S, Llewelyn MJ et al. BMJ 2019;364:l440. doi: 10.1136/bmj.l440
[15] Pasipanodya JG, Srivastava S & Gumbo T. Clin Infect Dis 2012;55(2):169–177. doi: 10.1093/cid/cis353
[16] Beardmore RE, Pena-Miller R, Gori F et al. Mol Biol Evol 2017;34(4):802–817. doi: 10.1093/molbev/msw292
[17] Takesue Y, Nakajima K, Ichiki K et al. J Hosp Infect 2010;75(1):28–32. doi: 10.1016/j.jhin.2009.11.022
[18] Smieszek T, Pouwels KB, Dolk FCK et al. J Antimicrob Chemother 2018;73(Suppl 2):ii36–ii43. doi: 10.1093/jac/dkx500
You may also be interested in
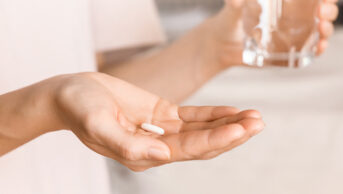
Pharmacists provide antibiotics only where clinically appropriate, minor ailments scheme data show
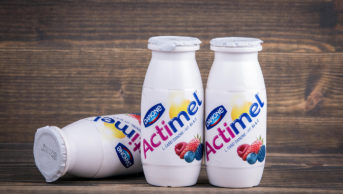
Probiotics should be considered when prescribing antibiotics, finds systematic review
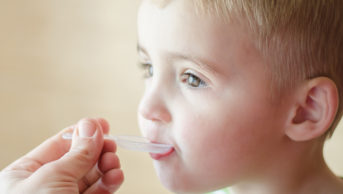