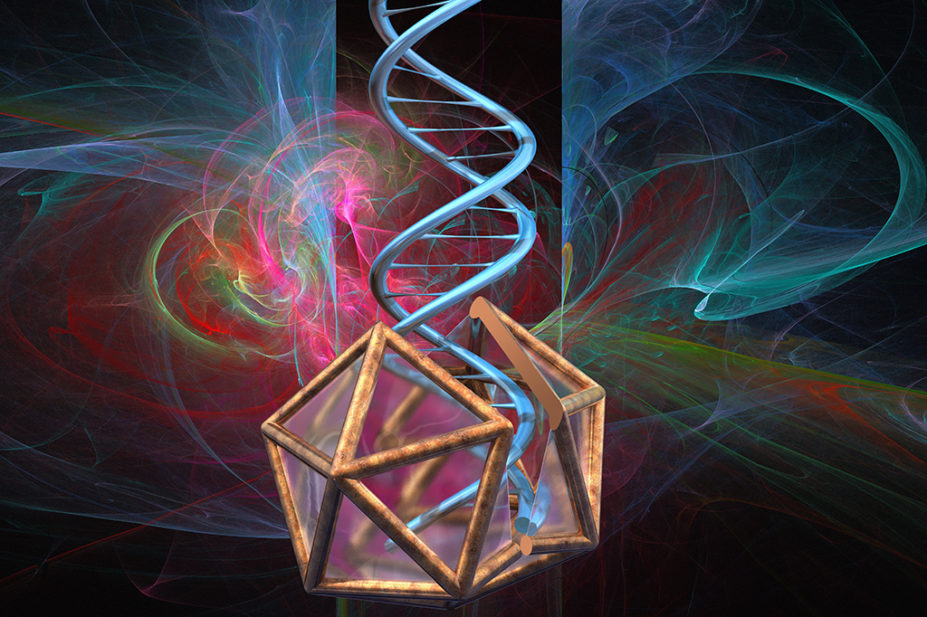
LAGUNA DESIGN/SCIENCE PHOTO LIBRARY
In 1999, the gene therapy field was rocked by the death of an 18-year-old clinical trial participant, Jesse Gelsinger.
Gelsinger suffered from ornithine transcarbamylase deficiency (OTCD), an X-linked genetic disease resulting from a mutation in the OTC gene, which leads to a build up of ammonia in the liver and blood.
Gelsinger was the 18th trial participant to receive the gene therapy — which aimed to inject working copies of the OTC gene by way of a viral vector — but just four days after receiving it he suffered a catastrophic immune reaction and died from multi-organ failure, leading to the suspension of gene therapy trials for around a decade[1].
But now, two decades later, in vivo gene therapy is finally making its way back. The majority of advanced therapy medicinal product (ATMP) trials are currently investigating viral-vector-mediated gene therapies, with an even distribution between in vivo and ex vivo gene delivery methods.
In the past five years, we’re really gaining some traction
Anne Black, regional quality assurance specialist pharmacist and chair of the Pan UK Pharmacy Working Group for ATMPs
“In the past five years, we’re really [gaining] some traction,” says Anne Black, regional quality assurance specialist pharmacist and chair of the Pan UK Pharmacy Working Group for ATMPs.
Gene therapy uses sections of DNA — usually genes — to treat or prevent diseases that are caused by the absence of a particular protein. In many cases, this involves adding DNA that contains a functional version of the missing or faulty gene responsible for making the protein.
In contrast to ex vivo therapies — such as chimeric antigen receptor (CAR) T-cell therapy, which involves the genetic modification of cells outside of the body and subsequent transplantation into patients — in vivo gene therapies require a vector (usually a virus) to transport the required genetic sequence into the patient’s cells (see Figure).
This same viral vector technology has been used in the development of several effective COVID-19 vaccines, including the one manufactured by AstraZeneca/Oxford University.
Both gene therapy and the COVID-19 viral vector vaccines take advantage of the virus’ ability to enter a cell.
In the case of the COVID-19 vaccines, the virus is engineered with the genetic code for the spike, or ‘S’, protein of the SARS-CoV-2 virus and is able to hijack the cell’s protein-making machinery to produce this antigen, so the body can mount an immune response against it.
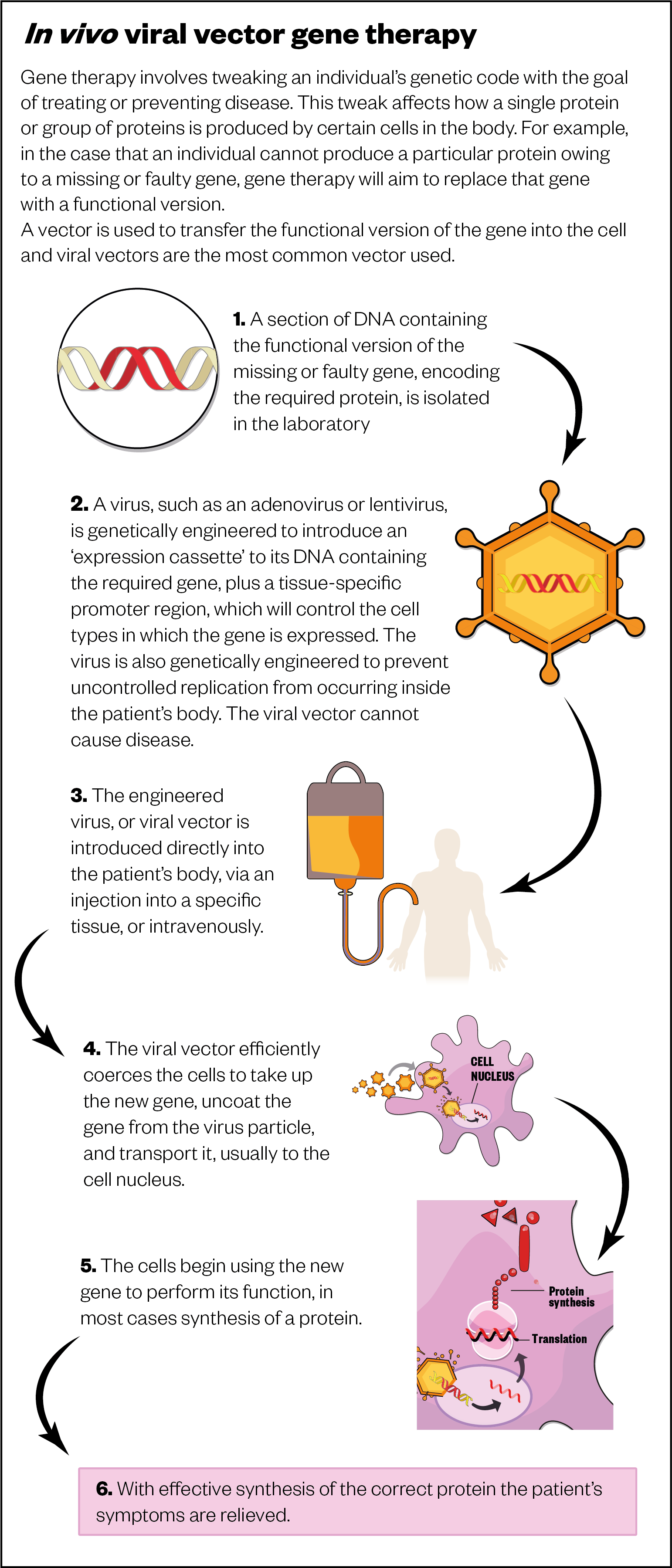
Emergence of in vivo gene therapies
However, the idea of delivering the gene via engineered viral vectors has been around for more than 30 years. The first time it was used successfully was in 1984, when scientists at the Boston Children’s Hospital, Massachusetts, used a virus to insert genes into blood-forming stem cells.
Glybera (Alipogene tiparvovec; uniQure biopharma B.V.) — the first in vivo gene therapy approved by the European Medicines Agency (EMA) in 2012 — was designed to treat lipoprotein lipase deficiency, a rare disease that prevents the breakdown of fats leading to abdominal pain and sometimes severe pancreatitis. At a cost of more than £700,000 per treatment, Glybera was the world’s most expensive treatment at the time and was only ever given to one patient in Germany before being withdrawn in 2017 owing to its commercial failure.
In the UK, the first gene therapy to become available was Luxturna (voretigene neparvovec; Novartis), which was approved in 2018 at a cost of £613,410 per patient at full price. The first of a number of ophthalmic gene therapies in development, Luxturna works by stopping the progression of sight loss resulting from inherited retinal dystrophy, caused by mutations in the RPE65 gene, which produces an enzyme necessary for retinal cells to function[2].
There have been other approvals since Luxturna’s, most recently Zolgensma (onasemnogene abeparvovec; Novartis), a one-time infusion to treat infants with spinal muscular atrophy, which received a conditional marketing authorisation in May 2020[3]. At £1.8m per single dose, Zolgensma now holds the accolade of the world’s most expensive drug; a five-month old baby became the first patient to receive it in May 2021.
A number of in vivo virus-based gene therapy approvals are expected in the coming years, with research spanning a vast number of therapeutic areas, including ophthalmology, haematology and metabolic diseases, as well as oncology, neuromuscular, neurological and cardiovascular diseases.
Challenges
Uta Griesenbach, who runs a gene therapy research group at Imperial College London, says that it has been “fantastically rewarding” to see the success stories coming through and new treatments being licensed.
However, the rollout of gene therapy has not been without significant challenges and the current burgeoning pipeline of gene therapies was not a foregone conclusion.
The most notable obstacle faced in the gene therapy field was that of Gelsinger in 1999, who is understood to have died after his body overreacted to the adenovirus vector. Gelsinger had a rare disorder in which the liver lacks a functional copy of the ornithine transcarbamylase (OTC) gene and, consequently, the body is unable to eliminate ammonia, a toxic breakdown product of protein metabolism.
In the clinical trial he was involved in, a weakened adenovirus had been engineered to deliver a functioning copy of the OTC gene into the liver. The trial was a safety study, aimed at moving toward a treatment for babies with the same genetic disease as Gelsinger, and was not intended to improve the participants’ health; but, despite this, Gelsinger was keen to be involved[1].
Several patients in earlier cohorts had suffered side effects, including some cases of severe liver reactions, which later raised questions from both scientists and the FDA as to why Gelsinger was treated at all.
Another hard lesson learnt through gene therapy trials was that of insertional mutagenesis, which occurs when the DNA sequence that is introduced integrates itself within the genome of the host organism, resulting in deregulation of genes around the insertion site.
A gene therapy for children with severe combined immunodeficiency was incredibly successful … but a small proportion developed leukaemia induced by the vector
Uta Griesenbach, Imperial College London
“A gene therapy for children with severe combined immunodeficiency (SCID) was delivered [to two independent groups in London and Paris] and was incredibly successful,” explains Griesenbach. “But, [in 2008; between three and six years later], a small proportion of children [in Paris] developed leukaemia induced by the vector, which had inserted itself into a gene that controls cell division[4].”
Since these events, Mimoun Azzouz, a neuroscientist at the University of Sheffield, says that “substantial refinements” of the viral vectors used in gene therapy have made current gene therapies safer and more efficient.
Azzouz heads up the university’s Gene Therapy Innovation and Manufacturing Centre (GTIMC), one of three pioneering UK hubs announced in March 2021 and funded by the Medical Research Council (MRC), Biotechnology and Biological Sciences Research Council (BBSRC) and medical charity, LifeArc[5].
Adeno-associated virus vectors
The earlier gene therapies used adenovirus vectors, owing to their ease of production and high levels of gene expression, but attention has now shifted to adeno-associated virus vectors (AAVs). These small 20nm viruses are unable to replicate independently without the presence of other viruses, meaning they cannot alone cause disease or extreme immune responses, making them safer to use.
In gene therapies, the engineered viruses contain an ’expression cassette’ added to their DNA that consists of the therapeutic gene, plus a tissue-specific promoter region, which will control the cell types in which the gene is expressed. Many gene therapies only need to be delivered once and will continue to function for long periods.
However, even with improved vectors, delivery of gene therapy to some locations in the body is easier than others. For example, retinal cells are long-lived and do not divide, so once delivered by injection the therapy will continue to act for a long time; this explains the large number of ophthalmic therapies in development.
“The blood-ocular barrier prevents the widespread dissemination of locally administered gene therapies throughout the body, potentially reducing the risk of off-target effects or systemic side effects,” explains Charlotte Arnold, vice president of corporate affairs at Gyroscope Therapeutics, a biotechnology company based in both the UK and United States.
Gyroscope is currently running phase II clinical trials for its AAV vector gene therapy GT005, to treat geographic atrophy (GA), which occurs as part of late-stage age-related macular degeneration (AMD). It has reported positive interim trial data, showing that GT005 was well tolerated. In the majority of participants, GT005 therapy resulted in increased levels of the missing protein, complement factor I (CFI), which helps regulate immune responses in the eye. The company anticipates completion of the trial in the next three years[6].
Another gene therapy company, MeiraGTx, is soon to enter phase III trials to treat X-linked retinitis pigmentosa, caused by the loss of the retinitis pigmentosa GTPase regulator (RPGR) gene. MeiraGTx is working with Janssen Pharmaceuticals to take this and further ocular gene therapies to market; but its gene therapies are not restricted to the eye.
We have a programme for Parkinson’s disease, delivering a small local dose to a specific area in the brain called the subthalamic nucleus
Alexandria Forbes, chief executive officer at MeiraGTx
“We have a programme for Parkinson’s disease, delivering a small local dose to a specific area in the brain called the subthalamic nucleus,” says Alexandria Forbes, chief executive officer at MeiraGTx. This region is hyperactive in individuals with Parkinson’s disease owing to an absence of dopamine, which the gene therapy aims to restore. So far, two completed phase II studies have shown promising results.
However, despite these successes, the questions arising from early gene therapy failures still remain and have led to significant caution among regulators, as well as those developing gene therapies.
For example, sales of the gene therapy, Zynteglo, which is used to treat the blood disorder, beta-thalassaemia, were voluntarily suspended in February 2021 after its manufacturer, Bluebird Bio, notified the EMA that bb1111 — the investigational therapy for patients with sickle cell disease, which uses the same technology — may have been linked to a case of acute myeloid leukaemia (AML)[7]. However, sales resumed in July 2021 after the EMA concluded that there is no evidence that Zynteglo causes AML. The company also temporarily suspended its phase I/phase II trial (HGB-206) and phase III trial (HGB-210) of bb1111 but these have now resumed.
The next generation
In general, the gene therapies currently on the market have tolerable side effects that can often be treated with steroids, but one unresolved issue is whether a patient’s antibody immune response may itself prevent the therapy from working. This was also a consideration in the development of the COVID-19 vaccine and why AstraZeneca and Oxford University decided to use a chimpanzee adenovirus vector, rather than a human one, in the development of their vaccine.
“For some of the AAV serotypes, a large proportion of the population has pre-existing immunity, which is a problem,” explains Griesenbach. This will be caused by previous exposure to similar circulating viruses. “Antibodies will bind to the virus and then the vector can’t enter cells,” she adds.
To counter this, researchers are looking for alternative AAVs (see Table). “Maybe vectors that come from other species that humans don’t have immunity to,” suggests Griesenbach.
This issue has been a problem for Griesenbach’s work on gene therapies for lung diseases, such as cystic fibrosis, which should be a prime candidate for gene therapy as a single gene disorder.
What we’ve learned over the past 25 years is that the lung has very powerful defense mechanisms
Uta Griesenbach, Imperial College London
“But, what we’ve learned over the past 25 years is that the lung has very powerful defense mechanisms, extra and intracellular barriers that make efficient gene transfer to the lung more challenging,” she explains.
In addition to this, lung cells are renewed every 18 months so any gene therapy would need to be repeatedly administered and AAV vectors lose efficacy after one dose owing to the development of immunity.
However, a solution could be found in lentiviral vectors. These are retroviruses, like HIV, that are able to insert a copy of their genome into the host’s DNA. They have been engineered to deliver therapeutic genes multiple times without inducing an immune response, although it is not yet clear why.
“One hypothesis is that the lentivirus doesn’t have as many surface proteins as other viruses, another that the virus enters the cells very quickly,” says Griesenbach, who expects her lentiviral vector gene therapy for cystic fibrosis to move into clinical trials in the next few years[8].
Another future innovation which could widen the types of diseases treatable, is to combine gene therapies with small molecule drug ‘switches’.
MeiraGTx is developing such a system using synthetic segments of RNA, called riboswitches, based on a regulatory mechanism commonly found in bacteria. Adding instructions for these switches to the expression cassette means that a small molecule drug can be used to control the splicing of the therapeutic gene. Only with the small molecule present will the correct protein be made; in effect, acting as an on/off switch for the therapeutic gene.
Forbes hopes the method will enable gene therapies to be developed for conditions that require treatment with short-lived hormones or peptides. For example, MeriaGTx is trying to use the approach to deliver glucagon-like peptide-1 receptor agonists (GLP-1 RAs) to treat type 2 diabetes mellitus, which currently needs to be injected by patients twice a day.
“Rather than having to engineer those peptides, to make them non-natural [to increase their half-life], we can put the gene into the body, and literally switch them on with a pill when needed,” says Forbes.
From the laboratory to patients
The future promise of gene therapy is tantalising, but getting there will require new infrastructure, particularly in the manufacturing of viral vectors. The viral vector technology required for AstraZeneca/Oxford’s adenovirus-based COVID-19 vaccine drove the expansion of manufacturing capabilities in the UK; contract manufacturer Oxford Biomedica opened a new Oxford-based production suite in 2020 to produce the viral vectors used in the vaccine, adding an additional 1,000L of manufacturing capacity over 18 months, with further expansion expected.
“Gene therapy manufacturing is quite challenging,” says Azzouz. He explains that there are no standard methods and, for example, purifying a gene therapy product is likely to require bespoke chromatography columns that will need to be developed for each vector.
Gene therapy manufacturing is quite challenging
Mimoun Azzouz, neuroscientist, University of Sheffield
“The assays for assessing [gene therapy quality] are one of the main issues,” says Forbes. “You have to be able to show the [regulatory] agencies that every one of your batches is the same within the parameters you have laid out and these assays are complicated to develop, qualify and validate.”
Currently, the UK is in danger of falling behind in the race to develop commercial in vivo gene therapies.
“[There are] some excellent and promising gene therapy programmes emerging from UK universities, but these programmes are significantly delayed or fail altogether because of the lack of [gene therapy medicinal products (GTMP)] manufacturing facilities,” says Azzouz.
The March 2021 announcement of an £18m investment in three gene therapy manufacturing hubs in Sheffield, South Yorkshire; Bristol and London may put development back on track and address some of the challenges in manufacturing AAV vectors as well as training a new GTMP manufacturing workforce[9]. An additional government-funded GTMP manufacturing centre in Stevenage, Hertfordshire, is also hoped to bridge the gap between scientific research and full-scale commercialisation.
At the clinical end of the spectrum, three advanced therapy treatment centres (ATTC) — Midlands-Wales; iMatch in Manchester, Greater Manchester; and Northern Alliance in Newcastle, Tyne and Wear — are now operating within the NHS to bring gene therapies and other pioneering ATMPs to patients. As Azzouz points out: “We need to make sure that things are optimised at the other end as well, that the units that will deliver these specialised therapies are ready, patient preparation and follow up is ready, [and] pharmacists need to be trained to handle these single injections, including any special storage.”
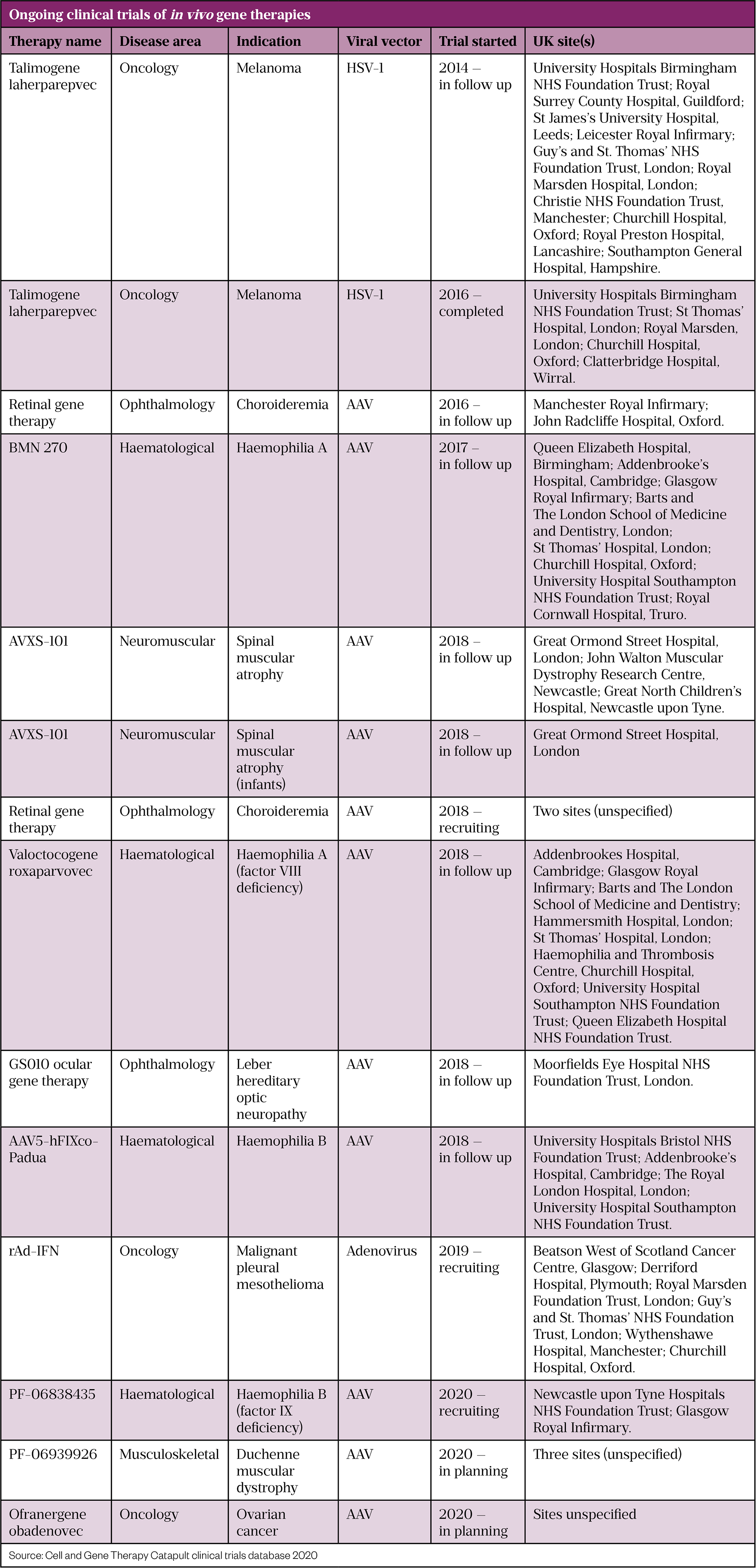
Training up pharmacists
Alongside Black, the Pan UK Pharmacy Working Group for ATMPs has been working hard to provide support for pharmacists in their knowledge of GTMPs. Established in 2018, the group has produced guidance for chief pharmacists in a ‘how to guide’ for organisations implementing in vivo gene therapies[10]. Black says pharmacies will have a large part to play in any roll out.
“In vivo gene therapies are not cellular products and, therefore, they can be handled and prepared within a pharmacy where stability allows. But the optimal place for preparation of a gene therapy needs to be decided through the governance process in the organisation. If this is outwith pharmacy, then pharmacy oversight is still required.”
Black recommends that in vivo gene therapy injections are prepared in an aseptic pharmacy environment[11].
“We would say that, where a preparation environment is not mandated by the [summary of product characteristics] or trial protocol, a negative pressure isolator within a positive pressure cascade suite is adequate preparation of many in vivo gene therapies but this does depend on the individual product.”
Negative pressure isolators or glove boxes are used for the safe handling of cytotoxic drugs to provide a barrier between operator and product, with transfer hatches to bring preparations into and out of the handling system. A positive pressure cascade suite provides a clean-room environment that uses airflow to limit contamination.
However, while most pharmacies preparing cytotoxic therapies could do this in principle with appropriate segregation, “it doesn’t mean that they have [spare] capacity if there was to be an explosion in marketed gene therapy products,” Black adds.
I am optimistic and confident that in the next five to ten years, we will see some wonderful therapies for some devastating disorders
Mimoun Azzouz, neuroscientist, University of Sheffield
In this eventuality there would need to be more investment in these facilities. A 2020 UK government report on pharmacy aseptic capacity argued for improvements in aseptic services to manage the current preparation of injectable medicines, before even adding provision for new gene therapies[12].
The introduction of significant numbers of in vivo gene therapies is also going to require wider training in the UK pharmacy community.
“We are working very closely with the ATTCs. They are putting together training modules, which are to be released and we’re certainly working on a specific one for pharmacy,” says Black.
For those working in the field, after many decades of failure, the future is finally looking bright.
“I am optimistic and confident that in the next five to ten years, we will see some wonderful therapies for some devastating disorders,” says Azzouz.
However, Griesembach does caution: “We have to be realistic and careful that we’re not over-hyping what gene therapy can do.”
These therapies are likely to be expensive, “[so] you need to pick disease indications that currently don’t have sufficient treatment options”. Nevertheless, she adds: “[It is] important to convey the significant excitement in the field at the moment.”
- 1Rinde M. The Death of Jesse Gelsinger, 20 Years Later. Distillations: using stories from science’s past to understand our world. 2019.https://www.sciencehistory.org/distillations/the-death-of-jesse-gelsinger-20-years-later (accessed 7 Sep 2021).
- 2Luxturna. European Medicines Agency. 2019.https://www.ema.europa.eu/en/medicines/human/EPAR/luxturna (accessed 7 Sep 2021).
- 3Zolgensma. European Medicines Agency . 2021.https://www.ema.europa.eu/en/medicines/human/EPAR/zolgensma#:~:text=Zolgensma%20received%20a%20conditional%20marketing,EU%20on%2018%20May%202020 (accessed 7 Sep 2021).
- 4Howe SJ, Mansour MR, Schwarzwaelder K, et al. Insertional mutagenesis combined with acquired somatic mutations causes leukemogenesis following gene therapy of SCID-X1 patients. J Clin Invest 2008;118:3143–50. doi:10.1172/jci35798
- 5New gene therapy innovation centre to advance scientific discoveries into life-changing treatments. The University of Sheffield. 2021.https://www.sheffield.ac.uk/news/new-gene-therapy-innovation-centre-advance-scientific-discoveries-life-changing-treatments (accessed 7 Sep 2021).
- 6Gyroscope Therapeutics Announces Positive Interim Data from Phase I/II FOCUS Trial of Investigational Gene Therapy GT005. Gyroscope: Vision for Life. 2021.https://www.gyroscopetx.com/gyroscope-therapeutics-announces-positive-interim-data-from-phase-i-ii-focus-trial-of-investigational-gene-therapy-gt005/ (accessed 7 Sep 2021).
- 7Precautionary marketing suspension of thalassaemia medicine Zynteglo . European Medicines Agency. 2021.https://www.ema.europa.eu/en/news/precautionary-marketing-suspension-thalassaemia-medicine-zynteglo (accessed 7 Sep 2021).
- 8Alton EWFW, Beekman JM, Boyd AC, et al. Preparation for a first-in-man lentivirus trial in patients with cystic fibrosis. Thorax 2016;72:137–47. doi:10.1136/thoraxjnl-2016-208406
- 9Gene therapy innovation hubs launched. UK Research and Innovation. 2021.https://www.ukri.org/news/gene-therapy-innovation-hubs-launched/ (accessed 7 Sep 2021).
- 10Pharmacy Institutional Readiness for In-vivo (virus based) Gene Therapy Medicinal Products. Pan UK Pharmacy Working Group for ATMPs 2020. https://www.sps.nhs.uk/wp-content/uploads/2020/07/Pharmacy-Institutional-Readiness-for-in-vivo-virus-based-Gene-Therapy-Medicinal-Products-V1-July-2020.pdf (accessed 7 Sep 2021).
- 11Gene Therapy Medicinal Products. Pan UK Pharmacy Working Group for ATMPs 2019. https://www.sps.nhs.uk/wp-content/uploads/2019/09/PAN-UK-PWG-for-ATMPs-Gene-Therapy-Guidance-issue-2.pdf (accessed 7 Sep 2021).
- 12Transforming NHS Pharmacy Aseptic Services in England. Department of Health and Social Care 2020. https://assets.publishing.service.gov.uk/government/uploads/system/uploads/attachment_data/file/931195/aseptic-pharmacy.pdf (accessed 7 Sep 2021).