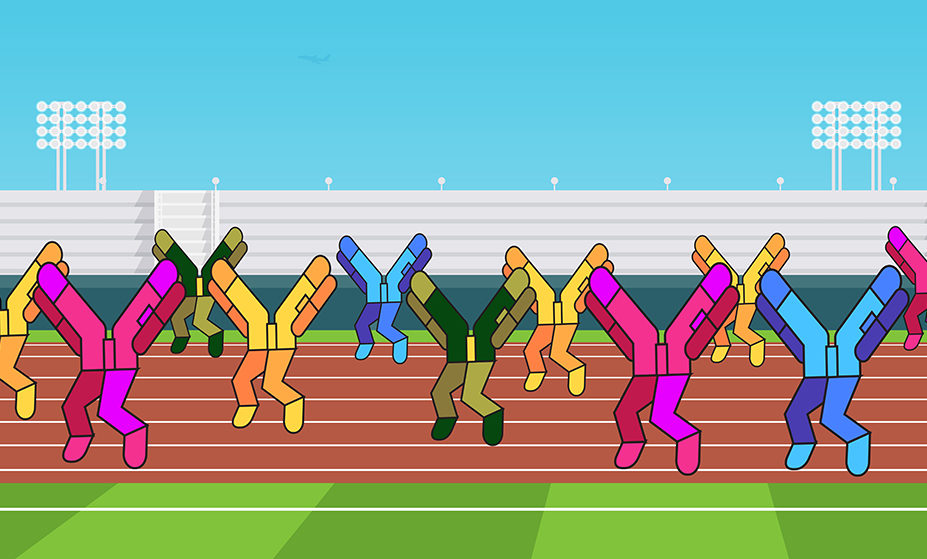
Mclean/Shutterstock.com
For SARS-CoV-2, change is the only constant. Now, 19 months into the COVID-19 pandemic, the virus continues to thwart us with variants: during winter 2020, the UK experienced a wave of the alpha variant, before another wave the following summer of the extremely transmissible delta variant. In all, four strains are currently denoted as “variants of concern” and others are being monitored.
Amid these viral spin-offs, vaccines tuned to the original version of SARS-CoV-2 have worked remarkably well at keeping people alive — so far. But they are not as effective at limiting transmission, particularly when it comes to the delta variant[1]. This infectiousness, combined with the social and logistical problems of quickly distributing vaccines to enough people, creates a crucible for viral mutation and the creation of yet more variants.
If we could do this, then we wouldn’t be constantly having to tweak the vaccines
Penny Ward, chair of the education and standards committee for the Faculty of Pharmaceutical Medicine
This dilemma leaves vaccine manufacturers scrambling to play catch up to the latest variant of SARS-CoV-2. To avoid an inefficient and costly ‘whack-a-mole’ approach of developing vaccines against each new strain, scientists are working on designing vaccines that work well against all SARS-CoV-2 variants, including those yet to emerge.
“If we could do this, then we wouldn’t be constantly having to tweak the vaccines,” says Penny Ward, chair of the education and standards committee for the Faculty of Pharmaceutical Medicine, a charity and professional membership body striving to advance the science and practice of pharmaceutical medicine. “It’s really about future-proofing.”
Developing a universal coronavirus vaccine that protects against current and future SARS-CoV-2 variants would not only help contain the current pandemic but may also provide a path to protection against other types of coronaviruses.
Preparing for the unknown
Coronaviruses are grouped into four genera, and only one of these — the beta-coronaviruses — contain viruses known to infect humans. Some cause mild colds, but three — SARS-CoV-2, SARS-CoV-1, and MERS-CoV — can be lethal. All are studded with the now infamous ‘spike’ protein, which binds to host cells to trigger infection.
Momentum for a universal coronavirus vaccine is building quickly, and the required tools are already at hand. Most efforts focus on the spike protein, aiming to produce ‘cross-neutralising’ antibodies that can tackle the spike, regardless of its source. Vaccination could help train the immune system to recognise differences as well as similarities in spike proteins found among the SARS-CoV-2 variants and, possibly, other beta-coronaviruses. Some approaches explicitly provide a diverse range of spike protein targets in the vaccine, whereas some are finding that even a monoculture of SARS-CoV-2 spike protein can elicit protection against other beta-coronaviruses. Other approaches are seeking to recruit T cell responses in addition to antibodies.
These experimental vaccines have produced signs of protection against SARS-CoV-2 variants and related beta-coronaviruses in rodents and monkeys. Multiple human clinical trials are underway to test these vaccines against SARS-CoV-2 and its variants.
The current COVID-19 pandemic caught us unawares
Charles Bangham, co-director of the Institute of Infection at Imperial College London
The results are emerging amid intensified calls for pandemic preparedness[2].
“The next pandemic is an unknown,” says Charles Bangham, co-director of the Institute of Infection at Imperial College London and an expert on viral immunology. “The current COVID-19 pandemic caught us unawares because everyone was expecting an influenza one. This shows that we can’t predict what’s coming next.”
Preparing for the next pandemic will require global coordination of scientists and policymakers, as well as governmental commitments to pay attention to potential viral threats. This will involve installing systems for rapid surveillance – as is already done with influenza – testing and contact tracing, and sequencing for molecular epidemiology.
“We don’t need another round of postmortems after the current pandemic to know what should be done,” adds Bangham. “Most of the lessons were learned in the previous epidemics, such as H1N1 flu.”
Mining for antibodies
The spike protein that studs coronaviruses is a complex structure, with a stem and head shape resembling a drumstick. The stem anchors the protein into the viral nucleocapsid, while the head contains the regions that are important for binding to the angiotensin-converting enzyme 2 (ACE2) receptor displayed by host cells, through which the virus gains entry to wreak its replicative havoc (see Figure).
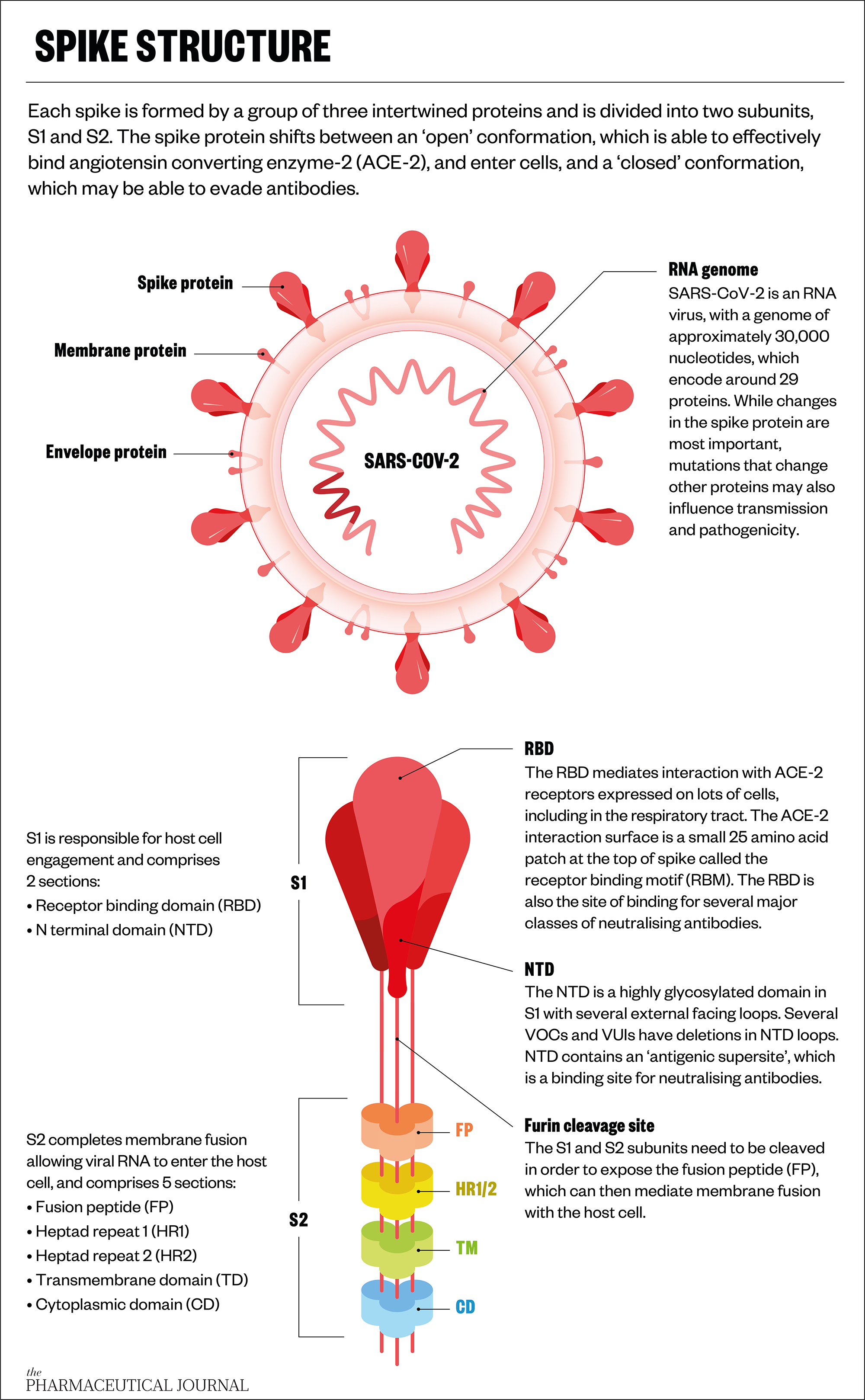
Up close, spike protein structures comprise a landscape of peaks and valleys. These vary between different coronaviruses and different variants, but some of these features are also conserved; universal coronavirus vaccines seek to exploit both.
Human immune responses sometimes stumble upon conserved features on their own. For example, survivors of the SARS-CoV-1 epidemic that emerged in China, spreading to four other countries, in 2003 have antibodies that can neutralise the spike protein from SARS-CoV-2. Likewise, mining antibodies from SARS-CoV-2 survivors revealed that some cross-react with spike proteins from multiple variants, and from different beta-coronaviruses that either infect humans or have the potential to[3,4].
Although these cross-neutralising antibodies tend to be rare among the gamut produced by the immune system, they point to an Achilles heel worth exploiting for vaccines or therapeutics.
These findings also show that training the immune system to attack one virus can generate antibodies that target others. A similar discovery befell Kevin Saunders, associate director of research at Duke University, North Carolina, while developing a booster vaccine for SARS-CoV-2[5].
Saunders and colleagues were focused on generating antibodies to the receptor-binding domain (RBD) of the spike protein from SARS-CoV-2, with the aim of blocking host cell infection.
Think of it as being like Velcro; if you engage a thousand loops and thousand hooks, it becomes a pretty strong interaction
Kevin Saunders, associate director of research at Duke University, North Carolina, United States
To elicit a strong immune response, they displayed 24 copies of the RBD protein on the surface of a nanoparticle, in a pattern that resembled their arrangement on coronaviruses, and added an immune cell-boosting adjuvant. The repeating geometry of RBDs turned out to be particularly good at activating immune cells that bind to pathogenic proteins — compared with an mRNA control that encoded just one RBD encased in a lipid bubble, similar to the current mRNA COVID-19 vaccines.
“I tell people to think of it as [being] like Velcro,” Saunders says of the RBD-nanoparticle array. “With Velcro, if a single hook and a single loop come together, it’s not very strong. But when you engage a thousand loops and a thousand hooks, it becomes a pretty strong interaction.”
Vaccinating monkeys with the RBD-nanoparticle elicited exceptionally high titers — or levels — of SARS-CoV-2 neutralising antibodies that interfered with the spike proteins’ ability to bind to ACE2 receptors — a good sign for a booster shot. Even better, the team also detected another antibody that could neutralise three SARS-CoV-2 variants (alpha, beta and gamma), as well as SARS-CoV-1, and two related bat coronaviruses that do not yet infect humans.
“Not only did we get really high neutralising antibody titers, but those antibodies were targeting a site on the receptor-binding domain that was conserved across multiple different coronaviruses,” says Saunders.
The reason for this finding remains unclear. The boosted immune response may have increased the number of antibodies, including the rare cross-neutralising antibodies, or it might be that, by stabilising the RBD protein onto the nanoparticle, it helped to reveal the parts of the antigen most readily recognised by the immune system.
Similar nanoparticle vaccines are already being tested in humans for protection against SARS-CoV-2. One, developed by Novavax, has been deemed safe in humans, and its recent phase III study reported strong protection against the original SARS-CoV-2 strain and the alpha variant among participants in the UK, with waning protection against the beta variant in a trial located in South Africa[6,7]. Another has begun testing in a phase I trial in the United States[8].
Mosaics and chimeras
Other efforts seek to train the immune system to recognise diverse targets representative of different strains of SARS-CoV-2 and other coronaviruses. This is a time-tested approach used in existing vaccines, such as the meningococcal and influenza vaccines.
This strategy met some success when tested in mice. A group at the California Institute of Technology in the United States developed “mosaic” nanoparticles that display the RBDs from four to eight different viruses from the sarbecovirus subgroup of beta-coronaviruses, which contains SARS-CoV-2, SARS-CoV-1, and some animal viruses that do not yet infect humans[9].
In February 2021, the group reported that mice immunised with these mosaic nanoparticles elicited neutralising antibodies to different viruses — both those where the virus’s RBDs were displayed on the immunising mosaic and those that were not. Similarly, in September 2021, another group reported a mosaic vaccine that protected mice from SARS-CoV challenge, even when the SARS-CoV RBD was not part of the vaccine[10].
Another vaccine approach relies on engineered spike chimeras, which are not found in nature. The chimeras are composed of three domains derived from different sarbecoviruses; for example, one chimera sported an RBD from SARS-CoV-1; an N-terminal domain from HKU3-1, a bat virus; and the rest from a spike protein from SARS-CoV-2. These chimeras were encoded as mRNA and delivered to mice, which then elicited high levels of broadly protective neutralising antibodies against sarbecoviruses, including SARS-CoV-2 alpha and beta variants[11]. Again, some of the antibodies recognised viruses that were not part of the immunising chimera.
Yet there is a limit to how many different parts of a virus can be contained in any one vaccine, says Saunders, who is also a co-author of the paper on the chimera approach.
“For the best vaccine, I think you’re going to have to have a mixture of the two types of ideas. You still need the antibodies to focus in on what’s conserved, not just what is strain-specific,” he says.
Trying for T cells
Biotech company Inovio is trying to combine both approaches in its design of a DNA-based vaccine for SARS-CoV-2. The company has an algorithm that analyses reported genome sequences of SARS-CoV-2 to identify conserved and divergent parts, which are then used to construct a synthetic spike sequence. This designer spike is encoded as DNA, which is more stable than mRNA and so can be stored at room temperature for up to a year — an advantage for distributing vaccines worldwide.
“It incorporates all of those aspects of the variants that are known around the globe at the moment into one molecule,” says Kate Broderick, senior vice president of research and development at Inovio.
Preliminary results show that mice vaccinated with one of these vaccines, called INO-4802, produce neutralising antibodies to the original SARS-CoV-2 virus, and to its alpha, beta, and gamma variants[12].
Another vaccine —INO-4800 — is based on the first few documented SARS-CoV-2 sequences. This shows some promise against SARS-CoV-2 variants, and a phase II/III trial in the United States should be completed by September 2022[13,14].
Antibodies are such amazing molecules that are so sensitive and so selective
Kate Broderick, senior vice president of research and development at Inovio
Both Inovio vaccines elicited potent T cell responses in mice and, for INO-4800, also in humans. T cell responses are harder to measure than antibodies, and so are often overlooked in measures of immunity. But T cells represent another front against disease because they can be trained to kill infected cells, as well as to boost the activity of antibody-making B cells. In addition, T cell responses may be more durable than antibody levels and may not be easily thwarted by the mutations that inevitably occur in the spike protein during viral replication.
“Antibodies are such amazing molecules that are so sensitive and so selective, so they are really much more impacted by these kinds of mutational changes,” says Broderick. “Whereas T cells are less susceptible to a base pair change here and there.”
Similarly, biotech company Scancell has developed a DNA vaccine that targets the highly conserved nucleocapsid of the virus, in addition to the spike protein[15]. And, in September 2021, US biotech company Gritstone launched a UK trial of its self-amplifying RNA vaccine, which targets both spike and non-spike proteins.
Although the new vaccines in clinical trials will only test their mettle against SARS-CoV-2 and its variants, their broad protection in animals suggests they may offer some protection against other viruses. Vaccines against a broader group of viruses, such as beta-coronaviruses, will take longer to develop, but Bangham and others believe it is feasible.
“I would be confident that it is possible,” he says.
In the meantime, a focus on antibodies may also prove critical for therapies, such as monoclonal antibodies given to patients with COVID-19 to help them fight off the virus.
“There is still a pressing need for an antiviral arm to the therapeutic armamentarium against this virus,” says Ward, noting that both vaccines and antiviral therapies exist against influenza.
“So, while we’re fiddling around with the vaccines, we should not forget that we still need antivirals,” she adds.
- 1Eyre DW, Taylor D, Purver M, et al. The impact of SARS-CoV-2 vaccination on Alpha & Delta variant transmission. 2021. doi:10.1101/2021.09.28.21264260
- 2Why disciplines must work together to prepare for future pandemics. World Economic Forum. 2021.https://www.weforum.org/agenda/2021/07/disciplines-together-future-pandemics/ (accessed 28 Sep 2021).
- 3Wec AZ, Wrapp D, Herbert AS, et al. Broad neutralization of SARS-related viruses by human monoclonal antibodies. Science 2020;369:731–6. doi:10.1126/science.abc7424
- 4Tortorici MA, Czudnochowski N, Starr TN, et al. Broad sarbecovirus neutralization by a human monoclonal antibody. Nature 2021;597:103–8. doi:10.1038/s41586-021-03817-4
- 5Saunders KO, Lee E, Parks R, et al. Neutralizing antibody vaccine for pandemic and pre-emergent coronaviruses. Nature 2021;594:553–9. doi:10.1038/s41586-021-03594-0
- 6Heath PT, Galiza EP, Baxter DN, et al. Safety and Efficacy of NVX-CoV2373 Covid-19 Vaccine. N Engl J Med 2021;385:1172–83. doi:10.1056/nejmoa2107659
- 7Shinde V, Bhikha S, Hoosain Z, et al. Efficacy of NVX-CoV2373 Covid-19 Vaccine against the B.1.351 Variant. N Engl J Med 2021;384:1899–909. doi:10.1056/nejmoa2103055
- 8Phase 1 Clinical Trial of WRAIR-developed COVID-19 Vaccine Begins. Walter Reed Army Institute of Research. 2021.https://www.wrair.army.mil/node/636 (accessed 28 Sep 2021).
- 9Cohen AA, Gnanapragasam PNP, Lee YE, et al. Mosaic nanoparticles elicit cross-reactive immune responses to zoonotic coronaviruses in mice. Science 2021;371:735–41. doi:10.1126/science.abf6840
- 10Walls AC, Miranda MC, Schäfer A, et al. Elicitation of broadly protective sarbecovirus immunity by receptor-binding domain nanoparticle vaccines. Cell Published Online First: September 2021. doi:10.1016/j.cell.2021.09.015
- 11Martinez DR, Schäfer A, Leist SR, et al. Chimeric spike mRNA vaccines protect against Sarbecovirus challenge in mice. Science 2021;373:991–8. doi:10.1126/science.abi4506
- 12Reed CC, Schultheis K, Andrade VM, et al. Design, immunogenicity and efficacy of a Pan-SARS-CoV-2 synthetic DNA vaccine. 2021. doi:10.1101/2021.05.11.443592
- 13Tebas P, Yang S, Boyer JD, et al. Safety and immunogenicity of INO-4800 DNA vaccine against SARS-CoV-2: A preliminary report of an open-label, Phase 1 clinical trial. EClinicalMedicine 2021;31:100689. doi:10.1016/j.eclinm.2020.100689
- 14Safety, Immunogenicity, and Efficacy of INO-4800 for COVID-19 in Healthy Seronegative Adults at High Risk of SARS-CoV-2 Exposure. ClinicalTrials.gov. 2020.https://clinicaltrials.gov/ct2/show/NCT04642638 (accessed 28 Sep 2021).
- 15Brentville V, Vankemmelbeke M, Metheringham R, et al. A novel bivalent DNA vaccine encoding both spike protein receptor-binding domain and nucleocapsid protein of SARS-CoV-2 to elicit T cell and neutralising antibody responses that cross react with variants. 2021. doi:10.1101/2021.06.18.448932