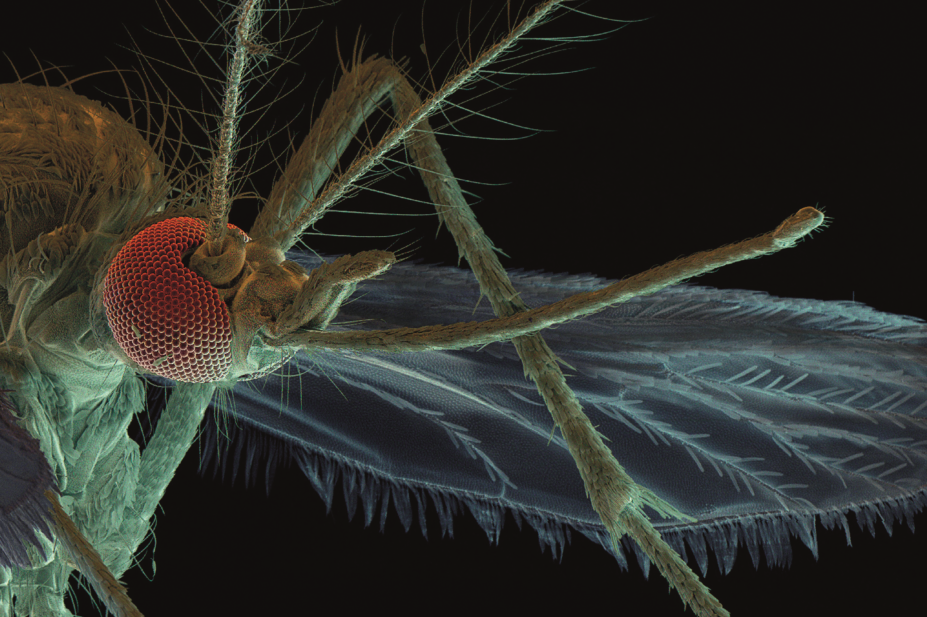
David Scharf / Science Photo Library
In the spring of 2015, a previously rare and little-known disease suddenly reared its head in northeastern Brazil. The illness had mild symptoms, including skin rash, fever and headaches, and it took several months for public health authorities to identify it as the obscure Zika virus.
The mosquito-borne Zika virus is a flavivirus, closely related to dengue, yellow fever and West Nile viruses. It was first identified in 1947 in Uganda, but there had been few recorded human cases until a large outbreak in 2007 on the island of Yap in Micronesia, in the western Pacific Ocean. Once the virus took hold in Brazil in 2015, it spread rapidly — in less than a year, cases were cropping up throughout South America and the Caribbean, and in parts of North America.
Then things got more complicated. Although primarily transmitted by mosquitos, it turned out that the virus could also be passed on sexually, and from pregnant women to their foetuses. And medical complications began cropping up, beyond the usually mild and nondescript symptoms of the disease. Brazil began reporting an increase in Guillain-Barré syndrome — a neurological disorder in which a person’s immune system attacks the peripheral nerves — in people with a history of Zika infection. There was also a disturbing rise in the number of babies born with microcephaly, which Brazilian doctors linked to Zika infections in the mothers. A study by the US Centers for Disease Control and Prevention found that as many as 10% of babies born to pregnant women with Zika infections had birth defects[1]
.
Little is known about the link between Zika and these neurological complications, or even their full extent, says Monica McArthur, an infectious disease researcher at the University of Maryland in Baltimore.
Source: Courtesy of Monica McArthur
Monica McArthur, an infectious disease researcher at the University of Maryland, says that the full spectrum of postnatal outcomes for infants born to mothers infected with Zika is not known
“We don’t really know the full spectrum of postnatal outcomes for infants born to mothers infected with Zika,” she says. “And we don’t know exactly how or why it causes neurological manifestations in adults, or what predisposes people to them.”
The immunology of Zika is also not well understood. “We’re still just starting to learn the more detailed immunological responses associated with Zika virus infection,” she added. “A lot of what we know is based on dengue virus and other flavivirus immunology.”
We’re still just starting to learn the more detailed immunological responses associated with Zika virus infection; a lot of what we know is based on dengue virus and other flavivirus immunology
People who are infected with Zika do develop antibodies against the virus, and researchers think that those neutralising antibodies are probably protective, but they do not know what level someone has to have, or if some other associated immune response is actually causing the protection, says McArthur. The other adaptive immune response, the T-cell response, is also present, but even less is known about that. “Helper T-cells and cytotoxic T-cells respond to Zika virus infection and are probably involved somehow in protection, but exactly what that role is, is still unclear,” she says.
Since the outbreak, researchers have made good progress in developing vaccines against the disease, with some candidates now in phase II trials. However, the brief nature of the outbreak is making wide-scale testing of the new vaccines difficult and some research programmes have already been curtailed.
Emergency response
By early 2016, the World Health Organization (WHO) was growing increasingly concerned about the Zika outbreak and its associated neurological complications. On 1 February 2016, the WHO declared it a Public Health Emergency of International Concern and launched a worldwide effort to coordinate the development of vaccines and treatments for the disease.
This was a virus not many people had heard of, so there was nothing in the pipeline; we made incredible progress to having vaccines in an advanced state of development in a short time
The research community was not really prepared to focus on such an obscure virus, but responded with surprising speed, says Rick Bright, director of the US Biomedical Advanced Research and Development Authority (BARDA). “This was a virus not many people had heard of, so there was nothing in the pipeline,” he says. “We made incredible progress to having vaccines in an advanced state of development in a short time.”
Source: Courtesy of Rick Bright
Rick Bright, director of the US Biomedical Advanced Research and Development Authority, says the research community made incredible progress to having vaccines in an advanced state of development in a short time
Annelise Wilder-Smith, an infectious disease researcher at the Lee Kong Chian School of Medicine, Singapore and a WHO consultant on Zika virus, says there are now around 45 vaccine candidates listed in the WHO’s pipeline tracker. Most are in preclinical development, with several in phase I clinical trials, and a few that have reached phase II.
The vaccines in development cover the whole range of vaccine types, from traditional live and inactivated virus vaccines to more experimental DNA, mRNA and protein subunit vaccines. “We want a diverse portfolio because we don’t know what kind of immune response will be needed,” says Bright. DNA and inactivated whole-virus vaccines are the types at the most advanced stage of development.
DNA and RNA vaccines have generated a lot of excitement, says Wilder-Smith, because they have the potential to be rapidly scaled-up and deployed during an outbreak should they prove to be efficacious. DNA vaccines work by creating a plasmid (a circle of DNA) that encodes the genes for the virus’s envelope proteins. Once this plasmid makes its way into the nucleus of the target cell, the cell’s own molecular machinery begins producing the viral proteins, which then induce an immune response. “The body’s own cells become the factory for these vaccines,” says Joseph Kim, chief executive of the Pennsylvania-based Inovio Pharmaceuticals, which is developing a DNA vaccine for Zika.
RNA vaccines work in much the same way, although the RNA can start producing viral proteins as soon as it enters the cell, without having to penetrate the nucleus. Massachusetts-based Moderna Therapeutics is developing two RNA vaccines for Zika, one of which is currently undergoing a phase I trial.
DNA and RNA vaccines have several potential advantages — a major one being speed. The process does not require access to the actual virus — just its genetic sequence — to design the synthetic proteins. “Genetic information collected in the field can be uploaded into the Cloud, and we can start making a vaccine within days,” says Kim.
Genetic information collected in the field can be uploaded into the Cloud, and we can start making a vaccine within days
Inovio’s Zika vaccine went from concept to first human dose in just seven months, which Kim believes is the fastest any vaccine has been put into practice in history. The company published the results of its phase I trial of the vaccine in October 2017 — in which 100% of participants produced antibodies to the virus after three doses of vaccine — and has begun phase II trials[2]
. The US National Institutes of Allergy and Infectious Disease (NIAID) is also developing a DNA vaccine for Zika, which is now in phase II trials.
DNA and RNA vaccines are also designed to be safer than conventional vaccines, says Kim, since there is no possibility of being exposed to the disease, as is the case with live and inactivated virus vaccines. And they do not require any of the chemicals, preservatives or adjuvants that can occasionally cause problems with conventional vaccines. Conventional vaccines also need to be kept frozen, a major logistical headache in warm climates and developing countries — DNA vaccines, in contrast, can be kept in a simple refrigerator or even left at room temperature, says Kim.
Source: National Institute of Allergy and Infectious Diseases
An investigational DNA vaccine against Zika
But there are drawbacks. Getting the plasmid all the way into the nucleus of the target cells adds an extra step to the vaccination process. Inovio uses a pen-like instrument to deliver a mild electric pulse to open up the cells so that the DNA can get inside before injecting the vaccine — a process called electroporation. This increases the cost of the vaccine, although Kim says this can be driven down in mass production.
A larger hurdle is the fact that as yet, no DNA or RNA vaccine has been approved for use in humans for any disease, making companies and regulators more wary of the technology.
That’s one reason why the Osaka-based pharmaceutical company Takeda chose to work on a whole inactivated virus vaccine. “We considered which technology would be most appropriate for the Zika challenge,” says Gary Dubin, head of the global medical office in Takeda’s vaccine unit. “To address an emerging public health problem, we wanted to use the one most likely to succeed.”
Source: Takeda
Gary Dubin, head of the global medical office in Takeda’s vaccine unit, says Takeda’s whole-virus approach is “relatively straightforward”, using the same technology used in other flavivirus vaccines
Dubin says Takeda’s whole-virus approach is “relatively straightforward”, using the same technology used in other flavivirus vaccines. The virus is inactivated so that it cannot replicate, but it still produces immunity. Besides being based on an established and trusted technology, one advantage of whole-virus vaccines is that they include all parts of the virus, not just the surface proteins, so have more options when it comes to generating antibodies, says McArthur.
But they are slower to develop. Takeda’s vaccine only started its phase I trial in November 2017, and a US Government whole inactivated vaccine, developed by NIAID and the Department of Defense, is just getting ready to start phase II trials.
Challenging road
Zika virus presents some particular challenges for vaccine development. One drawback faced by both inactivated-virus vaccines and DNA or RNA-based ones is that the immunity they produce does not last very long. You need to give multiple doses and boosters to get a reasonable length of protection. “In the middle of an outbreak, that’s fine,” says Mark Challberg, who oversees flavivirus research at NIAID. Vaccines that provide temporary protection are sufficient for emergency use.
But in the long term, he says, we will want a childhood vaccine to prevent congenital infections in later life. To get life-long immunity from a single dose, like with the vaccine for the yellow fever flavivirus, a live attenuated virus is needed. The trade-off, says McArthur, is that you are injecting people with a replicating virus. And given that the main target population for the vaccine during an outbreak will be pregnant women, it gets even trickier. Live-virus vaccines, although highly effective, can have side effects that could harm the foetus, and although inactivated vaccines are routinely given to pregnant women without mishap, new vaccines are not usually tested in pregnant women because of safety concerns. “Platforms that don’t involve a live virus are more attractive because giving those to pregnant women is less fraught,” says Challberg.
Platforms that don’t involve a live virus are more attractive because giving those to pregnant women is less fraught
That is another reason Takeda opted for an inactivated virus, says Dubin. “We felt any approach that used live virus would be more challenging,” he says.
The close relationship between flaviviruses, such as Zika and dengue, also presents challenges. Flaviviruses can sometimes cross-react — an infection with one of the four strains of dengue virus, for example, can make a subsequent infection with another strain more severe. The antibodies to one may not completely neutralise the other, and allow it to enter cells more easily. In addition, T-cells overreact, producing too many cytokines that cause dangerous inflammation. The result can be the more dangerous dengue haemorrhagic fever. “There is some concern that that Zika infections could make dengue infections worse, or vice-versa,” says McArthur. And since vaccines produce the same antibodies as an infection, they have the potential to cause the same cross-reactions.
Source: National Institute of Allergy and Infectious Diseases
Zika virus particles
So far there have been no reports of cross-reactions between Zika and dengue, or other flaviviruses, says McArthur, but pharmaceutical companies are proceeding with caution. Takeda’s phase I trial, for example, will have two steps. First, the vaccine will be tested for safety and immunogenicity in people who are flavivirus-naive; then it will be tested in those who have been primed by a previous flavivirus infection. “We will carefully monitor their safety as the study is conducted,” says Dubin.
But it turns out the trickiest thing about getting a Zika vaccine to market is that the outbreak just didn’t last long enough. By November 2016, the WHO ended the public health emergency, and the number of cases has fallen markedly since the height of the outbreak. That is good news for people in the affected areas, but it makes testing the new vaccines much harder. “Because the rates are declining it’s going to be difficult to do a phase III trial to prove efficacy,” says Challberg. There just are not enough infections out there to get good statistical data in a reasonable amount of time.
Because the rates are declining it’s going to be difficult to do a phase III trial to prove efficacy
Already the drop in infections is leading to some research programmes being curtailed. In September 2017, the French pharmaceutical company Sanofi announced that it would not go ahead with a phase III trial of the US Government inactivated-virus vaccine, after BARDA “de-scoped” the contract to focus on more limited goals.
BARDA’s Bright says there is a back-up plan to deal with the dearth of human subjects. Researchers can use animal models to test a vaccine’s immunogenicity and immune response and gain regulatory approval that way, then study it more extensively when human cases return. He says this approach has been used before for national security threats, such as anthrax and botulism — illnesses that are so rare that there is no opportunity to test treatments. “There is a precedent for using alternative regulatory pathways for vaccines so that they will be available in an emergency situation,” he says.
Figure 1: Geographical distribution of Zika
Source: World Health Organization. Data as of 12 December 2017
The geographical distribution of Zika virus has expanded globally, particularly since 2015 in the Americas. The latest data indicate that there is still ongoing transmission in 72 countries.
Wilder-Smith says the WHO is concerned about the potential loss in momentum in Zika vaccine development as the number of cases wanes, but she feels there is still enough interest and funding out there to ensure that something makes it to the finish line. And that could make all the difference for the next outbreak. “We want to keep the momentum, but we don’t need 45 vaccines. We just need at least one,” she says.
And a pause in an outbreak is no time to stop work on a vaccine, says Kim. “As global health experts have learned, there is usually a lull before the next round,” he says. “So we caution against taking our foot off the accelerator. These are the times to prepare.”
Treating Zika
Not all work on Zika has focused on producing a vaccine. Several research groups are also looking for drugs to treat the disease.
Brian Geiss, a microbiologist at Colorado State University in Fort Collins, has been studying flaviviruses for 10 years. He knew that developing new drugs is expensive and can take a long time. “So I was wondering if something already on the market could work for Zika,” he says.
Source: Courtesy of Brian Geiss
Brian Geiss, a microbiologist at Colorado State University, believes sofosbuvir is a good candidate for phase III trials in humans, but says the price will have to come down before trials are feasible
Luckily, the US Food and Drug Administration recently approved a new drug for the flavivirus hepatitis C (HCV), sofosbuvir (Sovaldi; Gilead). Sofosbuvir targets the viral DNA polymerase to stop replication, and Geiss thought the polymerases in HCV and Zika might be closely related enough for it to work on both.
He tested sofosbuvir against several strains of Zika from around the world in cell cultures and in mice, and it worked well, reducing infections without increasing cell death and protecting the mice from lethal infections[3]
. The drug is already known to be safe in humans and is not thought to affect the foetus in pregnant women, so Geiss believes it is a good candidate for phase III trials in humans. It could even be used off-label for Zika, at the discretion of doctors or public health officials, he suggests. The downside is it is quite expensive — the cost will have to come down before clinical trials are feasible, says Geiss.
Like Geiss, Alysson Muotri, a molecular biologist at the University of California, San Diego, spotted the potential for sofosbuvir to work on Zika. He found that it blocked transmission from mother to baby in a mouse model[4]
. Muotri’s team also took a more wide-ranging approach to their search for treatments, and began screening a huge library of 10,000 already-approved drugs more or less at random. “We didn’t have a strategy, but by chance it worked,” he says. He quickly came up with a promising candidate: chloroquine, an anti-malaria drug. In mice it helped animals survive longer and reduced viral load[5]
.
Source: Courtesy of Alysson Muotri
Alysson Muotri, a molecular biologist at the University of California, and his team are screening a huge library of 10,000 already-approved drugs in their search for a Zika treatment
Muotri isn’t sure how chloroquine works on Zika. “As far as we can tell, probably it has something to do with autophagy [an intracellular degradation system], since chloroquine protects this pathway,” he says. “But we haven’t proved it.”
A big advantage with chloroquine is that it is quite cheap, so a public health system could treat an entire country with a small budget. And it is easy to administer. In the 1950s Brazil mixed it in with salt distributed in the Amazon to help prevent malaria.
Shelton Bradrick, a molecular biologist at the University of Texas in Galveston, also screened a small library of around 800 FDA-approved drugs and found 20 possible candidates for treating Zika, including antiparasitic drugs like ivermectin, and antibiotics like daptomycin, which could reduce the rate of infection in cell cultures[6]
. With the number of Zika cases on the wane, Bradrick is now testing his candidates against other flaviviruses, like yellow fever.
Reading this article counts towards your CPD
You can use the following forms to record your learning and action points from this article from Pharmaceutical Journal Publications.
Your CPD module results are stored against your account here at The Pharmaceutical Journal. You must be registered and logged into the site to do this. To review your module results, go to the ‘My Account’ tab and then ‘My CPD’.
Any training, learning or development activities that you undertake for CPD can also be recorded as evidence as part of your RPS Faculty practice-based portfolio when preparing for Faculty membership. To start your RPS Faculty journey today, access the portfolio and tools at www.rpharms.com/Faculty
If your learning was planned in advance, please click:
If your learning was spontaneous, please click:
References
[1] Reynolds MR, Jones AM, Petersen EE et al. Vital signs: update on Zika virus–associated birth defects and evaluation of all US infants with congenital zika virus exposure — US Zika pregnancy registry, 2016. MMWR Morb Mortal Wkly Rep 2017;66:366– 373. doi: 10.15585/mmwr.mm6613e1
[2] Tebas P, Roberts CC, Muthumani K et al. Safety and immunogenicity of an anti–Zika virus DNA vaccine — preliminary report. New Engl J Med, online 4 October 2017. doi: 10.1056/NEJMoa1708120
[3] Bullard-Feibelman KM, Govero J, Zhu Z et al. The FDA-approved drug sofosbuvir inhibits Zika virus infection. Antiviral Res 2017;137:134–140. doi: 10.1016/j.antiviral.2016.11.023
[4] Mesci P, Macia A, Moore SM et al. Blocking Zika virus vertical transmission. Sci Rep 2018;8:1218. doi: 10.1038/s41598-018-19526-4
[5] Shiryaev SA, Mesci P, Pinto A et al. Repurposing of the anti-malaria drug chloroquine for Zika virus treatment and prophylaxis. Sci Rep 2017;7:15771. doi: 10.1038/s41598-017-15467-6
[6] Barrows NJ, Campos RK, Powell ST et al. A screen of FDA-approved drugs for inhibitors of Zika virus infection. Cell Host Microbe 2016;20:259–270. doi: 10.1016/j.chom.2016.07.004