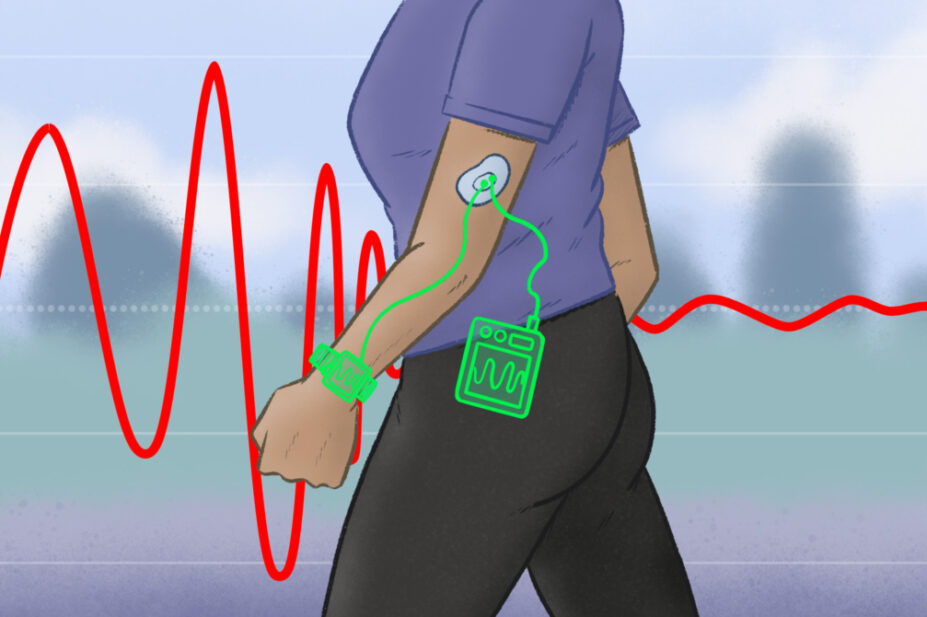
Wes Mountain/The Pharmaceutical Journal
The way in which prescribers approach the use of antibiotics has changed very little in the past few decades. They may be a bit more careful about when they deploy antibiotics, selecting for the right ones and trying to avoid overuse but, essentially, they prescribe a one-size-fits-all dose and hope for the best.
Yet there is plenty of evidence to suggest a more personalised approach would increase efficacy, reduce toxicity and help tackle antimicrobial resistance (AMR)[1]. Teams around the world are working on the best way to deliver this ‘precision dosing’ to achieve the perfect therapeutic concentrations.
Therapeutic drug monitoring (TDM) already does this to a certain extent and there is a growing global trend of using TDM in critically ill patients to track levels of drugs, such as vancomycin and aminoglycosides, where there is a narrow therapeutic window. However, increasingly intensive care units are also tracking concentrations of beta-lactam antibiotics, such as penicillins and cephalosporins. In 2015, a survey of centres around the world found only 6–7% were tracking beta-lactam levels, but a more recent study put the figure at 40%[2,3].
However, there are some major drawbacks to TDM. It requires access to a chromatography laboratory that can carry out the test, which is a complex process that demands careful calibration and validation. The result shows how much antibiotic a patient has in their bloodstream but it is likely to come two to three days after sampling, which may be too late to impact treatment. As a result, TDM for beta-lactams is not carried out routinely in the UK.
When we give everyone that one-size-fits-all dose, actually what happens is not everybody gets the same exposure
Richard Wilson, a research pharmacist at Imperial College London
But imagine if you could have real-time bedside monitoring of antibiotic levels — this is exactly the technology a team at Imperial College London is developing for use in patients with sepsis.
The team of biomedical engineering specialists has already completed the first human trial of a patch that can monitor levels of phenoxymethylpenicillin[4]. The idea came from the microneedle biosensors now commonly used to check blood glucose levels in people with diabetes, as did the concept of the next step — which the team is now working on — that uses the sensor to create a self-contained system to deliver the correct dose of antibiotics, in the same way that an artificial pancreas delivers insulin (see Figure).
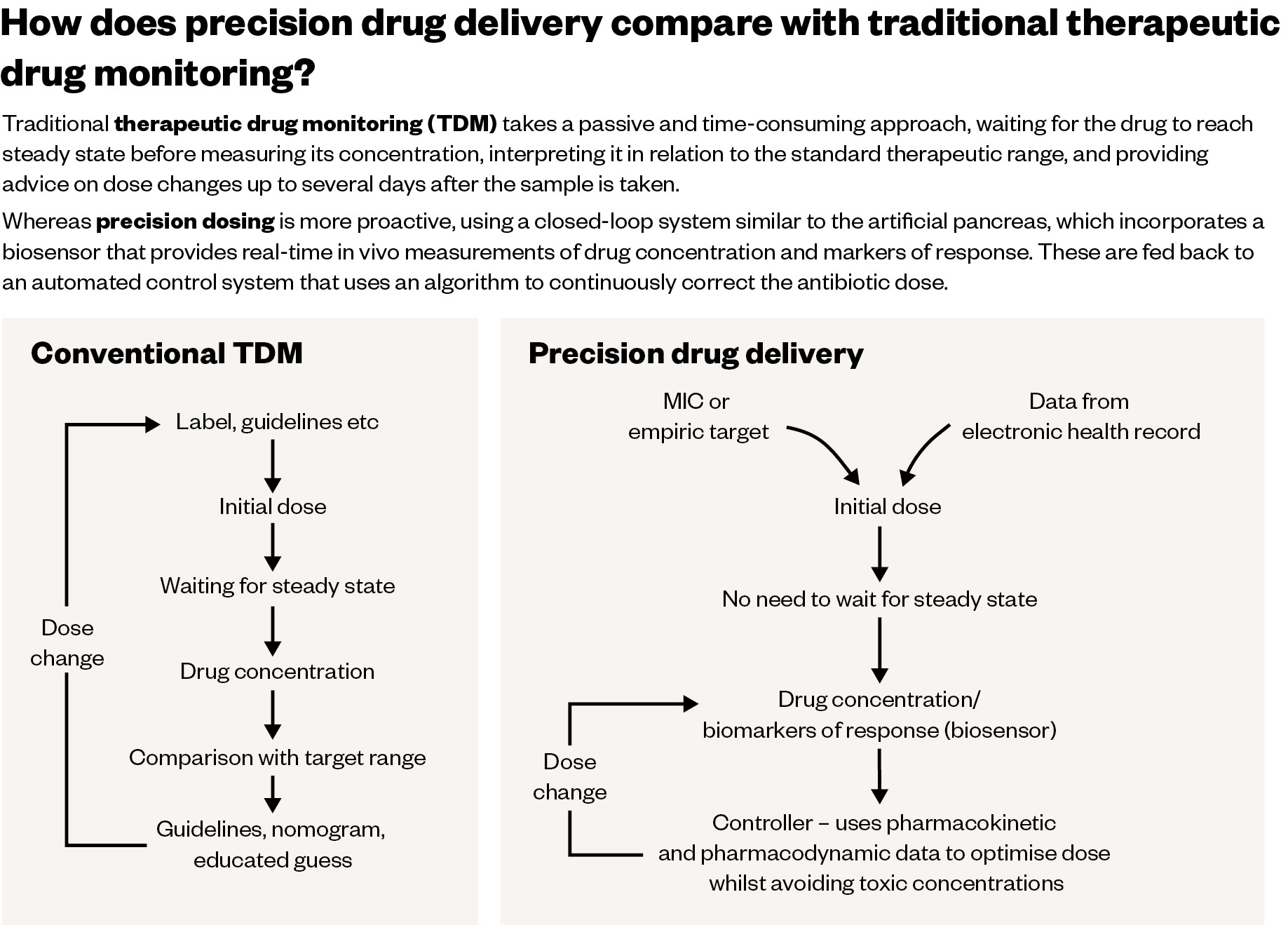
The Pharmaceutical Journal
Richard Wilson, a research pharmacist at Imperial College London, who specialises in both critical care medicine and infectious diseases, and works with the team creating the biosensors, says: “We’ve been in this space for maybe six or seven years and there’s very good data now coming out, especially from an intensive care setting, that when we give everyone that one-size-fits-all dose, actually what happens is not everybody gets the same exposure[5].
“Also, those suboptimal concentrations have been shown to correlate with a decreased chance of a successful outcome. There is also some work coming out around the subtherapeutic doses increasing the risk of antibiotic resistance developing within the population.”
Treatment failure
When thinking about precision dosing of antibiotics, there are several things that need to be considered when it comes to their concentration in the blood, explains Wilson. If the concentration is too high, it will likely induce toxicity, and if it is too low, it will not have any effect and increases the risk of selecting for a resistant population that spreads.
It may be as many as 50% of patients were not receiving what we would consider to be an optimal dose of drug
Jason Roberts, a research pharmacist at University of Queensland in Brisbane
Jason Roberts is a consultant clinical pharmacist and researcher with a similar background to Wilson who has spent years carrying out clinical trials to assess the benefits of monitoring antibiotic drug doses in critical care patients. Based at the University of Queensland in Brisbane, Australia, his team was one of the first to zone in on TDM for antibiotic use in critically ill patients.
A decade ago, the team did a large global study across 68 hospitals with more than 360 critically ill patients being treated with beta-lactam antibiotics. The results revealed that at least 16% were significantly underdosed and those patients were three times more likely to fail treatment[6].
“It may be as many as 50% of patients were not receiving what we would consider to be an optimal dose of drug,” explains Roberts. Since then, the team has done a lot of work looking at who is most high risk for treatment failure and why those drug concentrations in the body are too low to work. It found that, in short, most of the reasons relate to what else is happening in the body[7,8].
“Their illness and some of the treatments we try to use for the illness cause the problem,” says Roberts. “Essentially, if they have a bad response to the infection, they can’t maintain their own blood pressure, so we give them lots of intravenous fluids. If it’s a water-soluble [antibiotic] drug, it essentially dilutes the concentration [so it] is quite low in the early phase of treatment.
“But also, their response to all of this extra fluid is that they’re trying to perfuse all of their organs and their peripheries, and with that increased cardiac output, it just sends more drug through the kidneys per unit [of] time and it gets cleared a lot faster.”
It is a complex picture, further complicated by the fact that the pharmacokinetics are not just changing day by day, but most likely hour by hour. As in these seriously unwell patients there is a crucial early window for getting a therapeutic dose of antibiotic in place, standard TDM — at least, how the monitoring is currently performed in most hospitals that have access to it — is too slow[9].
This was exemplified by two trials, published in 2022, of individualised dosing of antibiotics, which found no benefit[10,11]. One study, conducted in eight hospitals in the Netherlands, involved adjusting doses of beta-lactam antibiotics and ciprofloxacin based on the monitoring of serum drug levels. But it took a while to get dose optimisation in place, perhaps missing that vital early treatment window, and there were also limitations to the approach, such as whether the dose adjustments were vigorous enough, says Roberts. The researchers concluded that, while the approach they took cannot be recommended, other ways of achieving an optimal dose should be studied.
Closed-loop control
The results of these two studies relate to another essential part of this puzzle, that Wilson and his team are keen to solve: what is the best body fluid to measure? Blood is the go-to because it is easily accessible — the cannulas are already there. But although blood is where you measure the infection, it is rarely the source.
To find out whether alternative fluids can be used in TDM, Wilson and his team have done some preliminary work looking at vancomycin levels in cerebrospinal fluid in patients with ventriculitis[12]. Other groups have looked at measuring drug concentrations in exhaled breath or urine, but these bodily fluids are all exuded by the body for a purpose, so are not ideal, explains Wilson. What he is most interested in is interstitial or tissue fluid.
“Tissue fluid is in equilibrium with the capillaries; just below the skin, you’ve got the interstitial space and that’s actually quite an easy compartment to access to do a procedure called microdialysis, which is an US Food and Drug Administration-approved procedure, and you can look at what drug concentrations are in there.”
What we’re looking at doing now is combining the measurements with closed-loop control
Richard Wilson, a research pharmacist at Imperial College London
“We’re a big multidisciplinary team and we saw the chemistry team were doing some work on looking at microneedles for glucose as part of the artificial pancreas project, and we thought this is going to be a great opportunity for antimicrobials as well.”
The technology works by coating a beta-lactamase enzyme on the microneedles, which is worn as a patch. The beta-lactam antibiotic comes into contact with the microneedles and is hydrolysed, causing a change in the local pH, which is detected electrochemically. A computer model then works out the drug concentration in the patient in real time — multiple times per second.
“What we’re looking at doing now is combining the measurements with closed-loop control. So, we published [a study] recently looking at this in silico [in computer simulations], using a computer algorithm that can correct your dose of antibiotics.[13]”
Other groups around the world, including Roberts’s team in Australia, are focusing on how to decide what concentration of a drug is effective. One potential target is to calculate the minimum inhibitory concentration (MIC), which tells you about the susceptibility or resistance of a bacteria to an antibiotic. However, this method involves growing cultures and there is a lot of potential variation in results. Another option is to identify a biomarker that tells you if the patient is responding.
“We’re very interested in looking at alternatives to MIC-based dosing. We have looked at what happens with your CRP [C-reactive protein] response. CRP is a very non-specific infection marker, but it is useful because it’s used regularly in routine practice in the UK,” says Wilson.
In addition to CRP, the team has also been investigating continuous monitoring of lactate, which can also be useful in patients with sepsis. The potential advantage of this approach — if it is shown to work — is that it is based on the patient’s response to an antibiotic rather than the bacteria itself.
Ultimately, the best solution is likely to bring together a range of information and use artificial intelligence to predict the risk of infection, the best antibiotic and the individual dose.
“We have designed the microneedles so we have four separate arrays — one as a control and three that can have different sensors,” says Wilson. “You could have a sensor that measures antimicrobial concentration, [one that looks] at some other biological molecule to see what the response is and feed all that into your closed-loop control and dose people from that.”
We have rapid diagnostic technology combined with a dosing software technology to help optimise a personalised approach to dosing
Jason Roberts, a research pharmacist at University of Queensland in Brisbane
Over in Australia, Roberts is leading the DIRECT study, in collaboration with colleagues in Italy[14]. The goal of the study is to combine rapid sequencing of pathogens from blood samples in patients with sepsis with personalised antibiotic dosing. If successful, the approach will help doctors to select the most appropriate antibiotic early at the start of treatment. Using dosing software developed by his team and daily sample testing, doctors should then be able to ensure the patient is getting enough of the drug they need.
The software is based on the concept that, from a pharmacokinetic point of view, we know a lot about antibiotics; for example, their half-life and how much binds to plasma proteins.
“In patients with sepsis, we have rapid diagnostic technology combined with a dosing software technology to help optimise a personalised approach to dosing,” explains Roberts. “It uses old TDM technology, not some of the newer technologies, but it’s something which we think is very worthwhile trying to test clinically. We’ve finished the first of our studies and we’re trying to progress that into a large clinical trial.”
What can hopefully be combined one day is the research on how personalised dosing works in practice in a real clinical population with the technology that can provide many data points on how the drugs behave in the body early on in the dosing.
“It will mean the first dose may not be perfect, but the second dose should be very, very close to optimal for what that individual patient needs,” says Roberts.
Computational intelligence
A team in the Netherlands has also developed software, called AutoKinetics, which takes information already in the patient’s electronic health record to help clinicians adjust antibiotic doses. The trial, published in 2022, showed that this personalised dosing approach — which has the benefit of being implemented as soon as the patient arrives in critical care — is safe and feasible[15].
It has never been really shown that adequate target attainment leads to better outcomes
Paul Elbers, a specialist in intensive care medicine at Amsterdam University Medical Centre
However, of the four antibiotics assessed, it was only when testing the software on ciprofloxacin that the team showed improved (and faster) attainment of the target plasma concentration. Clinical endpoints, such as mortality and length of stay in intensive care, were not significantly different, although that is not what the study was set up to look at, explains Paul Elbers, a specialist in intensive care medicine at Amsterdam University Medical Centre.
“One of the problems [in this area of research] is that it has never been really shown that adequate target attainment leads to better outcomes, although it is likely and there are many clues from the literature that it does, but [the evidence] is not rock solid.”
The clinicians who used the software gave positive feedback, he says, because, until then, they had not been aware that they might be underdosing patients. The exception to this was with meropenem, where the software, on average, suggested lowering the dose, which the clinicians were not comfortable doing.
“We are focused mainly on getting other models based on computational intelligence to the bedside related to pharmacokinetics, but this is a nice use case. We are now actively looking for a partner to maybe pursue this further,” he adds.
In the future, it may not just be those in critical care who could benefit from this personalised approach. Other patients who are likely to have poor outcomes; for example, patients with cancer who are immunosuppressed, or those with a medical device or prosthesis associated infection, could too.
The final piece of the jigsaw that should be considered is pharmacogenomics, says Roberts. “That’s not necessarily something which is as relevant for beta-lactam antibiotics, but for some of the triazole antifungal drugs, you can predict with a fair bit of accuracy how different the dosing requirements will be based on genotypic expression. That is a study that we’re looking to get under way at the moment.”
TDM is currently a reactive approach to correcting that initial dose, he says, but “the more relevant information you have at the start, the more proactive you are towards getting to an effective dose”.
- 1Rawson TM, Wilson RC, O’Hare D, et al. Optimizing antimicrobial use: challenges, advances and opportunities. Nat Rev Microbiol. 2021;19:747–58. doi:10.1038/s41579-021-00578-9
- 2Tabah A, De Waele J, Lipman J, et al. The ADMIN-ICU survey: a survey on antimicrobial dosing and monitoring in ICUs. J. Antimicrob. Chemother. 2015;70:2671–7. doi:10.1093/jac/dkv165
- 3Williams PG, Tabah A, Cotta MO, et al. International survey of antibiotic dosing and monitoring in adult intensive care units. Crit Care. 2023;27. doi:10.1186/s13054-023-04527-1
- 4Rawson TM, Gowers SAN, Freeman DME, et al. Microneedle biosensors for real-time, minimally invasive drug monitoring of phenoxymethylpenicillin: a first-in-human evaluation in healthy volunteers. The Lancet Digital Health. 2019;1:e335–43. doi:10.1016/s2589-7500(19)30131-1
- 5Onufrak NJ, Forrest A, Gonzalez D. Pharmacokinetic and Pharmacodynamic Principles of Anti-infective Dosing. Clinical Therapeutics. 2016;38:1930–47. doi:10.1016/j.clinthera.2016.06.015
- 6Roberts JA, Paul SK, Akova M, et al. DALI: Defining Antibiotic Levels in Intensive Care Unit Patients: Are Current -Lactam Antibiotic Doses Sufficient for Critically Ill Patients? Clinical Infectious Diseases. 2014;58:1072–83. doi:10.1093/cid/ciu027
- 7Setiawan E, Cotta MO, Abdul-Aziz M-H, et al. Population Pharmacokinetics and Dosing Simulations of Ampicillin and Sulbactam in Hospitalised Adult Patients. Clin Pharmacokinet. 2023;62:573–86. doi:10.1007/s40262-023-01219-5
- 8Shekar K, Abdul-Aziz MH, Cheng V, et al. Antimicrobial Exposures in Critically Ill Patients Receiving Extracorporeal Membrane Oxygenation. Am J Respir Crit Care Med. 2023;207:704–20. doi:10.1164/rccm.202207-1393oc
- 9Barreto EF, Chitre PN, Pine KH, et al. Why is the Implementation of Beta-Lactam Therapeutic Drug Monitoring for the Critically Ill Falling Short? A Multicenter Mixed-Methods Study. Therapeutic Drug Monitoring. 2023;Publish Ahead of Print. doi:10.1097/ftd.0000000000001059
- 10Hagel S, Bach F, Brenner T, et al. Effect of therapeutic drug monitoring-based dose optimization of piperacillin/tazobactam on sepsis-related organ dysfunction in patients with sepsis: a randomized controlled trial. Intensive Care Med. 2022;48:311–21. doi:10.1007/s00134-021-06609-6
- 11Ewoldt TMJ, Abdulla A, Rietdijk WJR, et al. Model-informed precision dosing of beta-lactam antibiotics and ciprofloxacin in critically ill patients: a multicentre randomised clinical trial. Intensive Care Med. 2022;48:1760–71. doi:10.1007/s00134-022-06921-9
- 12Arkell P, Wilson R, Watkins K, et al. Application of therapeutic drug monitoring to the treatment of bacterial central nervous system infection: a scoping review. Journal of Antimicrobial Chemotherapy. 2022;77:3408–13. doi:10.1093/jac/dkac332
- 13Herrero P, Wilson RC, Armiger R, et al. Closed-loop control of continuous piperacillin delivery: An in silico study. Front. Bioeng. Biotechnol. 2022;10. doi:10.3389/fbioe.2022.1015389
- 14Irwin AD, Coin LJM, Harris PNA, et al. Optimising Treatment Outcomes for Children and Adults Through Rapid Genome Sequencing of Sepsis Pathogens. A Study Protocol for a Prospective, Multi-Centre Trial (DIRECT). Front. Cell. Infect. Microbiol. 2021;11. doi:10.3389/fcimb.2021.667680
- 15Roggeveen LF, Guo T, Fleuren LM, et al. Right dose, right now: bedside, real-time, data-driven, and personalised antibiotic dosing in critically ill patients with sepsis or septic shock—a two-centre randomised clinical trial. Crit Care. 2022;26. doi:10.1186/s13054-022-04098-7