Stanford University bioengineer Stanley Qi was excited when the groundbreaking gene-editing CRISPR medicine Casgevy (exagamglogene autotemcel) made it to the market in late 2023. “I started working on CRISPR in 2012, and we didn’t even know if this system could work in human cells,” he says, adding that the speed with which the first medicine has been approved is “amazing”.
Casgevy, licensed for the blood disorders sickle cell disease and transfusion-dependent β-thalassemia, is likely to be the first of many CRISPR-based medicines. There are more than 85 registered clinical trials involving CRISPR, for conditions ranging from cancer and muscular dystrophies to rare genetic diseases, such as Rett syndrome.
Some of the therapies in trials are already using new technologies, developed with the hope of improving on the original CRISPR therapeutic concept. But there are still many challenges to overcome with the technique, including concerns about safety and efficacy.
What is CRISPR?
CRISPR — which stands for ‘clustered regularly interspaced short palindromic repeats’ — is a way of editing genes. The technique is borrowed from bacterial immune systems, which use an enzyme called ‘Cas9’ to chop up the genes of invading viruses, which are then saved as molecular mugshots.
The technique targets a specific DNA sequence, using a single strand of RNA as a guide, and uses the Cas9 enzyme to make a double strand cut in the DNA. When the cut is repaired naturally by the cells’ DNA repair mechanism, mutations are introduced that can permanently disrupt transcription of a gene, giving a potential therapeutic tool (see Figure 1). For discovering this technique, Emmanuelle Charpentier and Jennifer Doudna won the 2020 Nobel Prize in Chemistry.
Casgevy, from CRISPR Therapeutics and Vertex Pharmaceuticals, was approved by the Medicines and Healthcare products Regulatory Agency in the UK in November 2023 and approved by the US Food and Drug Administration (FDA) a month later. It treats sickle cell disease and transfusion-dependent β-thalassemia, which are caused by genetic mutations in the genes that produce beta-globin, leading to production of abnormal haemoglobin molecules that cause red blood cells to take on a crescent shape, causing severe pain and anaemia.
Rather than silencing the mutated gene, Casgevy switches off the BCL11A gene, which causes foetal haemoglobin to be produced, providing an adequate haemoglobin substitute. The treatment is administered by extracting a patient’s red blood cells, stem and progenitor cells from the bone marrow, and editing them using the CRISPR method before re-infusion.
By using an ex vivo approach, the Casgevy therapy bypasses the difficulty of delivering CRISPR editing molecules into cells. However, in vivo CRISPR therapies “have made tremendous progress over the past few years”, according to Ian Karp, senior vice president at genome-editing company Intellia Therapeutics, based in Cambridge, Massachusetts.
In November 2023, its leading in vivo CRISPR therapeutic NTLA-2001, which is being developed as a single-dose therapy in collaboration with Regeneron, began a pivotal phase III clinical trial to treat ATTR-CM, a rare and potentially fatal form of amyloidosis[1].
Off-target effects
Although companies are forging ahead with clinical trials, there have been some concerns about ‘off-target’ breaks in DNA strands caused by first-generation CRISPR technologies, where the strand is cut in a place that was not targeted by the guide RNA. This causes faulty repairs. Of particular concern are translocations, where detached fragments of different chromosomes become joined, which can lead to cancer[2].
Annarita Miccio, from the Imagine Institute in Paris — a research institute dedicated to genetic diseases — says that, in some ex vivo therapies, excessive off-target DNA damage ends up killing the edited cells, which can make it difficult to produce enough to infuse back into the patient.
Graphite Bio, based in San Francisco, California, halted its phase I/II CEDAR clinical trial of its sickle cell CRISPR therapy nulabeglogene autogedtemcel (nula-cel) in early 2023, after its first patients experienced prolonged low blood cell counts (pancytopenia)[3]. The ex vivo CRISPR therapy aimed to restore adult haemoglobin production by correcting a point mutation in the beta-globin gene. It is not known if the issue was linked to the sort of off-target damage Miccio describes, but shows that complications can arise.
Base editing CRISPR
A second generation CRISPR system — called ‘base editing’ — was first proposed in 2016, with the aim of reducing off-target effects. This uses an engineered Cas9 nickase enzyme, which makes only a single strand break in the DNA, fused with a base editing enzyme that can convert a cytosine (C) to a thymine (T) or an adenine (A) to a guanine (G) (see Figure 1).
It’s more like a pencil and eraser
Sekar Kathiresan, cardiologist and chief executive of Verve Therapeutics
Rather than a pair of scissors, “it’s more like a pencil and eraser”, explains Sekar Kathiresan, cardiologist and chief executive of Verve Therapeutics, based in Cambridge, Massachusetts, whose company is using the method.
Waseem Qasim, from UCL Great Ormond Street Institute of Child Health in London, has also been using this approach to produce an allogenic chimeric antigen receptor (CAR)-T cell therapy to treat children with a rare T-cell leukaemia. These patients do not have enough healthy T-cells for the established autologous CAR-T cell therapy, where a patient’s own cells are removed, modified to include a receptor that attacks the cancer cells and re-infused.
“We decided to try and engineer cells from healthy donors that could be used across multiple recipients, without the need for particular matching,” explains Qasim. His team has started using ex vivo CRISPR base editing to switch off three genes in the donor CAR-T cells, which will allow them to evade attack by the patients’ immune system.
“It’s surprisingly efficient. We can get it to work at certain [genomic] sites at almost 100% efficiency,” he says. In September 2023, his team reported interim results from dosing the first three children, and is moving forward with a further phase I trial for childhood acute lymphoblastic leukaemia[4].
But base editing has some drawbacks. If the mutated DNA base is neighboured by the same nucleotide, this will be edited too, known as bystander edits, which then adds an additional mutation that could have negative consequences. More crucially, not all diseases are caused by single mutations[5]. “For some diseases, the mutations are really well spread; for example, cystic fibrosis has more than 2,000 known mutations on a single gene,” explains Qi.
“To use this method, you’re going to need different [therapies] for every single mutation — that will be challenging.”
Prime editing CRISPR
Prime editing, introduced in 2019, is another second-generation CRISPR technique that partially overcomes the limitations of base editing by inserting small DNA stretches of up to 35 base pairs almost anywhere in the genome[6]. Here, instead of a base-editing enzyme, a modified Cas9 protein is fused to a reverse transcriptase enzyme, which can generate complementary DNA from an RNA template added to the RNA guide molecule. The prime editing guide (peg) RNA also has sequences that will wrap round and bind to the complementary DNA strand to be reverse transcribed. Although this creates two mismatched DNA strands, after the natural DNA repair process, a proportion of the molecules will include the corrected sequence (see Figure 2).
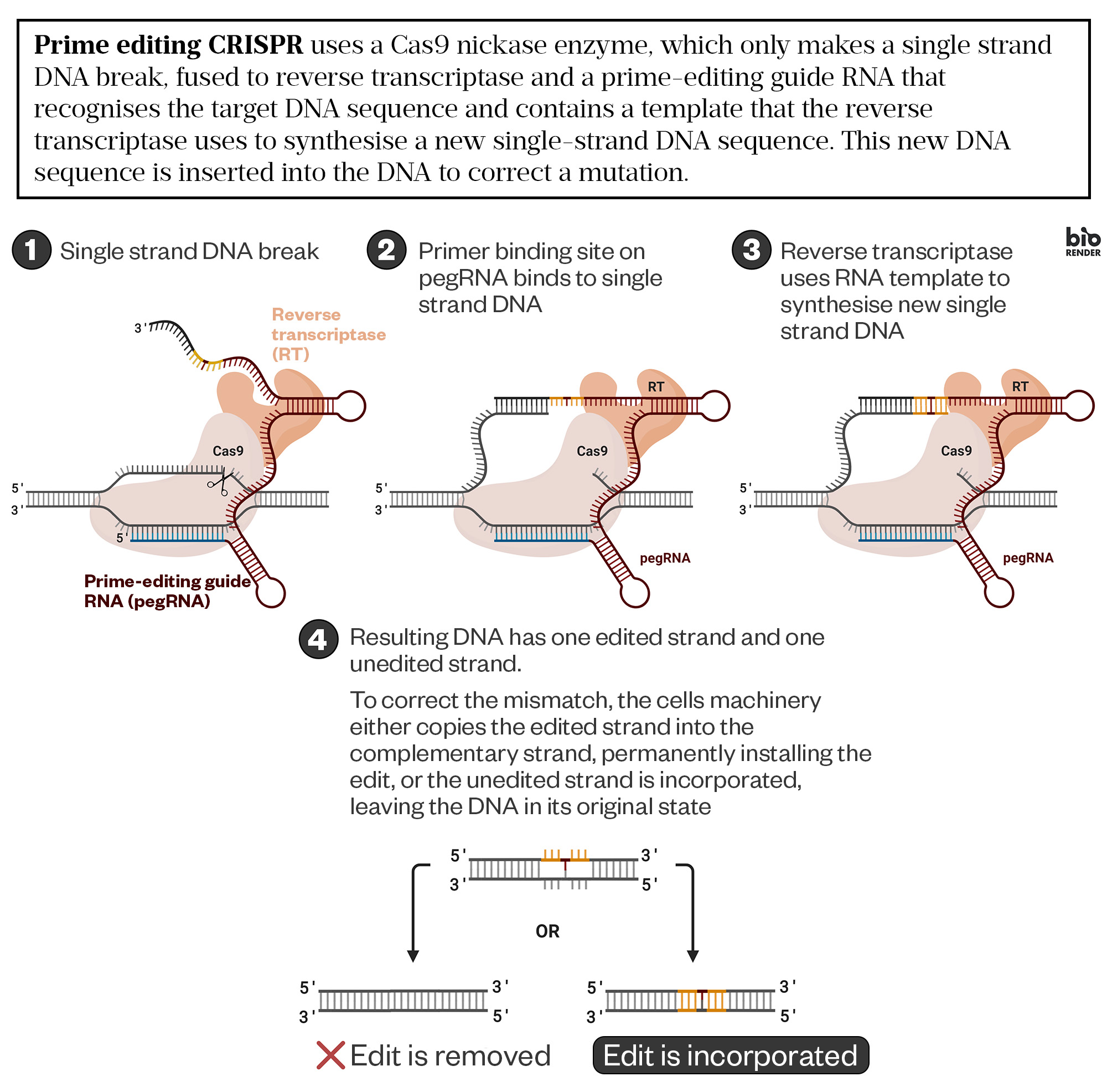
Ldinatto CC-BY-SA (adapted)
When prime editing came, this was really exciting
Patrick Harrison, a molecular biologist at University College
“When prime editing came, this was really exciting,” says Patrick Harrison, a molecular biologist at University College, Cork, Ireland, who has been developing a prime-editing therapy for cystic fibrosis (CF).
“If you had a disease where there’s two or three mutations very close together in a hotspot, you can potentially fix multiple mutations with the same peg RNA.” CF is often caused by clusters of mutations in the CFTR membrane protein, and its malfunction leads to a build-up of thick mucus, causing persistent lung infections. Harrison is working on a strategy that can correct two mutations in one therapy. Prime Medicine, a company based in Cambridge, Massachusetts, has 18 development programmes based on prime editing and, in January 2024, announced US$15m of support from the US Cystic Fibrosis Foundation to develop CF therapies.
Without introducing double strand breaks, both prime and base editing offer safer strategies with less likelihood of off-target mutations. But a recent comparative study showed that some breakages do still occur, leading to risks of genotoxicity[7]. “These events are much less frequent, but they still exist,” says Miccio. “You’re creating nicking and sometimes the repair system transforms this single strand nicking into double strand breaks.”
Epigenome editing
An alternative second-generation CRISPR technique that comes without these risks is epigenome editing, which does not modify the DNA code but modifies the methylation or histone structures that control gene expression, known as epigenetic marks. For example, where DNA is highly methylated, genes are usually switched off, but once methyl groups are removed, they are switched on. Using enzymes fused to the CRISPR location system could address multiple mutations by simply silencing a gene. Because the changes are only at the epigenome level, they are also reversible, with alternative enzymes delivered by CRISPR able to switch changes back without impacting the genetic code.
Qi’s biotech start-up Epic Bio, based in San Francisco, California, is using epigenome editing to tackle facioscapulohumeral muscular dystrophy, a genetic muscle disorder that currently has no cure, with patients often becoming wheelchair-bound by the age of 50 years. It is caused by a loss of methylation, leading to the abnormal expression of the DUX4 gene on chromosome 4, which is normally only expressed in the embryonic state. Epic Bio is using epigenome editing to re-methlyate the gene. The company has an investigational new drug authorisation for its first therapy and hopes to start a clinical trial in 2024.
A lot of epigenetic modifications are inherited
Stanley Qi, bioengineer, Stanford University
One question raised over epigenome editing is whether changes will be passed on or whether the cells will revert back to their original state when copied. “A lot of [epigenetic] modifications are inherited, so when cells divide, they not only copy their DNA codes, they also copy methylation patterns,” explains Qi, who thinks they may be a “one and done” therapy most of the time, although he says his team has observed that additional doses after several months produce step-wise improvements.
For many CRISPR therapies, a single dose seems to be sufficient and sometimes the only option. “If you use a viral vector [to deliver the CRISPR system], like the adeno-associated virus, you induce significant immunogenicity after the first dosing, making dosing a second time very challenging,” says Qi. The alternative is to deliver mRNA molecules using lipid nanoparticles (LPNs), rather than the Cas9 protein itself, in a system similar to the mRNA COVID-19 vaccine. “It’s much less toxic and immunogenic, and we also know from the COVID vaccine that people can receive multiple doses,” he says.
Targeted therapy
Targeted delivery — getting the CRISPR therapy to a particular organ or limiting the cell types that are edited — is one of the biggest problems remaining with in vivo CRISPR. Intellia Therapeutics has been using several delivery approaches, including non-viral LPNs that edit liver cells — the easiest organ to reach since molecules end up there owing to first-pass metabolism. “The next opportunity for us will be to expand the number of cell types that we can efficiently reach and edit within the human body,” says Karp. “We are continuing to work very hard on moving in vivo editing outside the liver to tissues such as bone marrow, the brain and muscles.”
In some ways, the science isn’t the challenge, it’s the bigger logistical issues
Patrick Harrison, a molecular biologist at University College
One idea to achieve this is to attach an antibody or antibody fragment that will home in on a specific cell type. Spotlight Therapeutics, based in Hayward, California, is one of the companies trying to do this. “We’ve taken a biologics approach,” says Mary Haak-Frendscho, chief executive of the company. “We deliver just the editor itself, rather than delivering it through a virus or through a highly engineered LPN.” The researchers bond the engineered Cas9 protein, preloaded with the guide RNA, to an antibody fragment and a cell-penetrating peptide — the combination ensures it gets to the right cells.
Intellia Therapeutics is also following a similar path, combining Regeneron’s antibody-targeted viral vector delivery technology with its CRISPR platform to target neurological and muscular diseases.
Blockbuster strategy
While CRISPR can be lifesaving, the cost associated with these therapies, particularly for relatively rare diseases, is prohibitively high. “In some ways, the science isn’t the challenge, it’s the bigger logistical issues of how do you get this to as many people as you can, as safely as you can, and yet still allow companies to be viable,” says Harrison.
The National Institute for Health and Care Excellence did not recommend Casgevy for use within the NHS in draft guidance, published on 14 March 2024. NICE said that the cost-effective estimate for Casgevy is higher than what NICE considers acceptable, even when using a higher cost-effective estimate than normal to account for health inequalities and the innovative nature of the technology. It added that approval would need collection of further data on its effectiveness as well as a commercial arrangement.
The answer may be to find a blockbuster CRISPR therapy. Verve Therapeutics is among several companies that think they have found one for the most common cause of death in the world — heart attacks. Verve’s one-time, intravenously-infused, base-editing CRISPR drug candidate VERVE-101 lowers cholesterol levels by turning off the gene that expresses the PCSK9 enzyme in the liver. People in which the gene is naturally turned off have a low risk of heart attack.
The company began a phase I trial of VERVE-101 in July 2022 and, in November 2023, released data for the first ten patients, who have familial hypercholesterolaemia (FH), which carries a high risk of heart attack[8]. “Initial durability data, just extending six months right now, is encouraging. The 55% reduction [in low-density-lipoprotein cholesterol] that we saw at two weeks after treatment in one patient has remained,” says Kathiresan. Investigators did, however, report elevated liver enzymes in some patients, suggesting the potential for liver damage, but Verve says the safety profile supports further development.
Further innovation
Although a second generation of CRISPR technologies is well on its way, innovations continue. One challenge is the large size of the Cas9 molecule, which makes it difficult to deliver and more toxic to cells. By a combination of mining the genomes of other microbes and engineering changes to the protein, Qi has developed an editing enzyme that is a third of the size of Cas9, called CasMINI[9]. Epic Bio is developing a highly compact epigenetic editor using this alternative Cas.
Delivery is also limiting the ability to insert longer stretches of genetic material, including whole genes, although several companies are trying. Prime Medicine is developing a CF therapy that would insert a sequence that has the potential to restore expression of the CFTR protein in all individuals’ lung cells, regardless of the underlying CFTR mutation.
What if you could put the working gene where the faulty gene is? That’s the promise
Sekar Kathiresan, cardiologist and chief executive of Verve Therapeutics
Being able to insert whole genes is the “end game”, says Kathiresan. “What if you could actually go right to the native DNA in the person, to where the faulty gene is, and essentially put the working gene where the faulty gene is? That’s the promise,” he says, adding that “it’s not super far away”.
Qi is confident that gene-editing therapies are here to stay, although which type of CRISPR method prevails, if any, is not yet clear. Qasim agrees, “whatever versions we’re using today, they might well get superseded quite quickly by newer, better, faster, more reliable versions”.
But Kathiresan says we should not forget how game changing CRISPR has already been. “The fact that you had the initial observation in 2012, and now, 11 years later, you have an FDA-approved product. For a brand-new technology, it’s unparalleled.”
- This article was amended on 15 March 2024 to remove information about a licensing application
- 1Intellia Therapeutics, Regeneron Pharmaceuticals. MAGNITUDE: A Phase 3 Study of NTLA-2001 in Participants With Transthyretin Amyloidosis With Cardiomyopathy (ATTR-CM). ClinicalTrials.gov. 2023. https://classic.clinicaltrials.gov/ct2/show/NCT06128629 (accessed 6 March 2024)
- 2Brunet E, Jasin M. Induction of Chromosomal Translocations with CRISPR-Cas9 and Other Nucleases: Understanding the Repair Mechanisms That Give Rise to Translocations. Advances in Experimental Medicine and Biology. 2018;15–25. https://doi.org/10.1007/978-981-13-0593-1_2
- 3Shyr DC, Lowsky R, Miller W, et al. One Year Follow-up on the First Patient Treated with Nula-Cel: An Autologous CRISPR/Cas9 Gene Corrected CD34+ Cell Product to Treat Sickle Cell Disease. Blood. 2023;142:5000–5000. https://doi.org/10.1182/blood-2023-188963
- 4Chiesa R, Georgiadis C, Syed F, et al. Base-Edited CAR7 T Cells for Relapsed T-Cell Acute Lymphoblastic Leukemia. N Engl J Med. 2023;389:899–910. https://doi.org/10.1056/nejmoa2300709
- 5Molinski SV, Shahani VM, Subramanian AS, et al. Comprehensive mapping of cystic fibrosis mutations to CFTR protein identifies mutation clusters and molecular docking predicts corrector binding site. Proteins. 2018;86:833–43. https://doi.org/10.1002/prot.25496
- 6Anzalone AV, Randolph PB, Davis JR, et al. Search-and-replace genome editing without double-strand breaks or donor DNA. Nature. 2019;576:149–57. https://doi.org/10.1038/s41586-019-1711-4
- 7Fiumara M, Ferrari S, Omer-Javed A, et al. Genotoxic effects of base and prime editing in human hematopoietic stem cells. Nat Biotechnol. 2023. https://doi.org/10.1038/s41587-023-01915-4
- 8Vafal S, Gladding P, Scott R. Safety and Pharmacodynamic Effects of VERVE-101. Verve Therapeutics. 2023. https://live-verve-therapeutics.pantheonsite.io/sites/default/files/2023-11/Verve_AHA_2023_LBS_for%20website.pdf (accessed 14 March 2024)
- 9Xu X, Chemparathy A, Zeng L, et al. Engineered miniature CRISPR-Cas system for mammalian genome regulation and editing. Molecular Cell. 2021;81:4333-4345.e4. https://doi.org/10.1016/j.molcel.2021.08.008