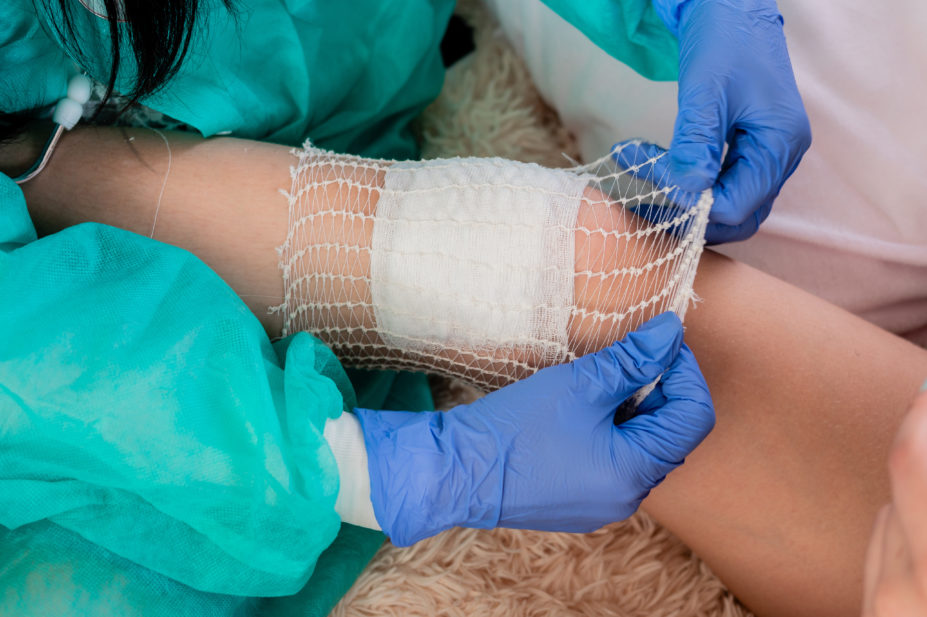
Shutterstock.com
After reading this article, you should be able to:
- Understand the difference between acute and chronic wounds;
- Explain the normal stages of wound healing and what can impair the process;
- Outline the therapies currently used to treat chronic wounds and new therapies in development.
A wound is any injury that disrupts the structure of healthy skin tissue caused by chemical, mechanical, biological or thermal trauma. Wounds can be classified as acute or chronic, depending on their period of healing[1]. Acute wounds usually heal without complication within ten days; however, chronic wounds do not undergo normal healing processes, commonly have exaggerated inflammation, persistent infections or microbial biofilm formation and persist longer than six weeks[2–4]. The most frequent causes of chronic wounds are pressure, diabetes and vascular diseases[5].
Chronic wounds are a global problem, with annual cases rising dramatically owing to the ageing population and increased prevalence of diabetes and obesity[6]. It is estimated that up to 7% of the UK adult population has a chronic wound, costing the NHS £8.3bn each year in staff costs, wound dressings and medication[7]. Individual costs for wound management have been reported to vary, from £358 to £4,684 per patient for a wound that follows the normal healing trajectory, increasing to £831 to £7,886 per patient for a chronic, non-healing wound[7]. The majority of the costs account for GP and nursing time, with infected wounds costing an additional £1.39bn on antibiotics[7].
Results from one study, published in 2020, found that 59% of chronic wounds healed if there was no evidence of infection, compared with 45% if infection was present or suspected[7]. Health conditions, such as diabetes mellitus and vascular disease, can predispose people to wounds that are difficult to heal, which can become chronic unless the underlying causes are addressed. For example, people with diabetes are prone to have a high incidence of wounds on their feet, which are slow to heal because of the impact of diabetes on the immune system, circulation and diabetic neuropathy. Complex chronic wounds, such as venous leg ulcers and diabetic ulcers, can significantly impact quality of life, morbidity and mortality[7].
Physiology of wound healing
Wound healing is a complex series of physiological reactions and interactions between numerous cell types and chemical mediators[8,9]. It comprises four coordinated and overlapping phases: haemostasis, inflammation, proliferation and remodelling[10].
The Figure below shows the phases of wound healing[11].
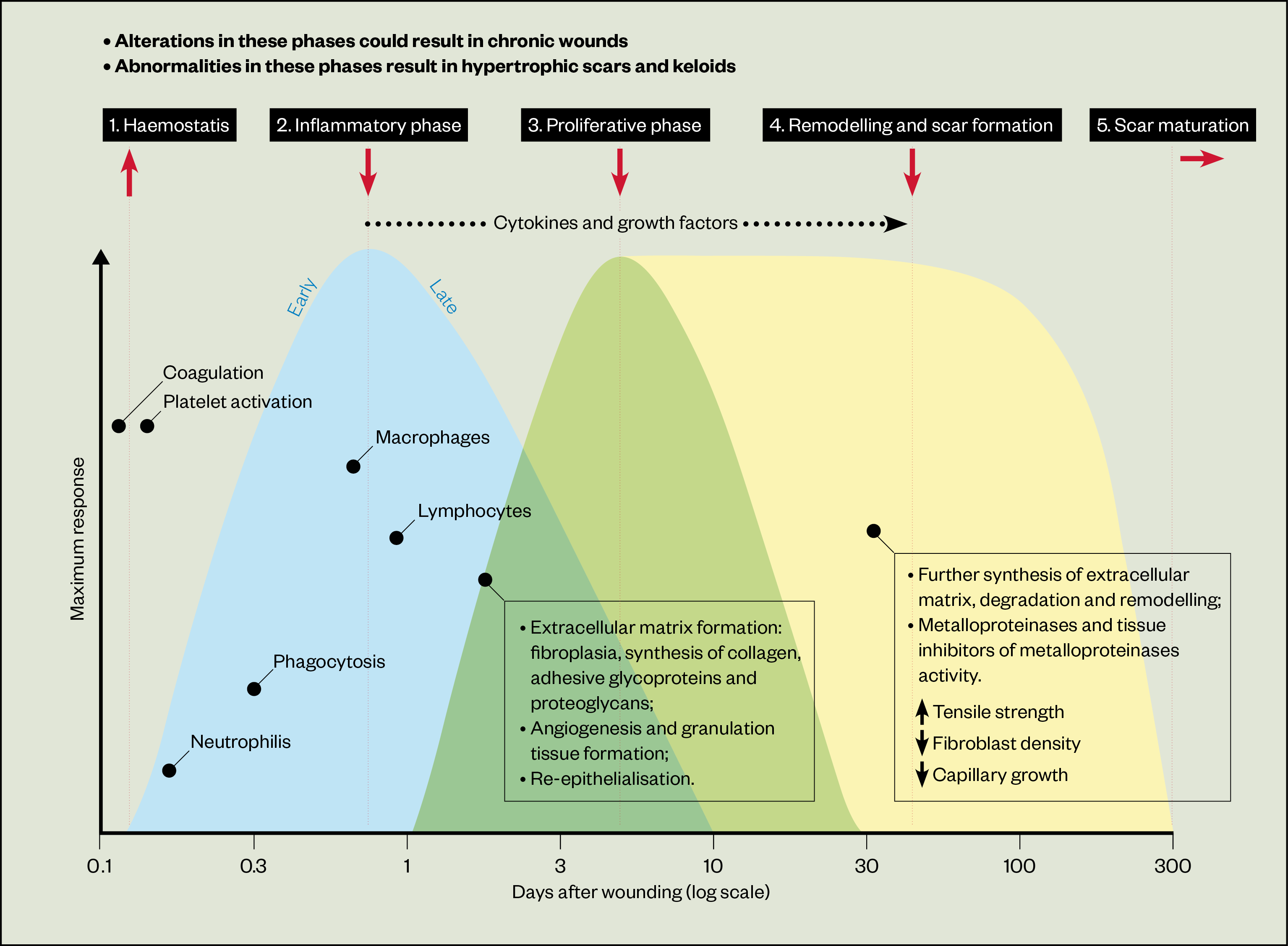
The first stage, haemostasis, is instantly activated after injury to stop bleeding at the site and prevent the entry of pathogens. In primary haemostasis, within seconds of an injury occurring, damaged blood vessels vasoconstrict to reduce blood flow through the wound area and diminish blood loss. Platelets adhere to the sub-endothelium of the impaired vessels, initiated by the presence of von Willebrand factor. This binds to glycoprotein Ib receptors on the surface of platelets, causing a conformational change on the platelet surface, activating platelets. These activated platelets release chemicals, such as adenosine diphosphate, serotonin and thromboxane A2, from their dense granules to stimulate platelet recruitment and adhesion to form a platelet plug[12,13]. Secondary haemostasis is a sequence of events, described as a ‘coagulation cascade’, that consequently converts soluble fibrinogen into insoluble fibrin. A fibrin mesh sticks to the platelet plug producing a haemostatic plug to seal the inside of wound[12,14].
At the beginning of the inflammatory phase, activated platelets also release pro-inflammatory cytokines and growth factors to stimulate the recruitment of immune cells to clean the wound area, initially involving infiltration of neutrophils and monocytes[15]. Monocytes undergo a phenotypic change to become macrophages. The previously constricted blood vessels also vasodilate because of increased prostaglandins, facilitating the chemotaxis of inflammatory cells[16,17]. The proliferation phase is charactered by re-epithelialization, capillary regeneration and the formation of granulation tissue (18). Fibroblasts and endothelial cells proliferate during this phase, stimulated by the numerous cytokines and growth factors released by the platelets and macrophages. This leads to the formation of new blood vessels in a process called angiogenesis[18].
After migration to the wound site, fibroblasts begin to proliferate and synthesize collagen and extracellular matrix components, such as proteoglycans, hyaluronic acid, glycosaminoglycans, and fibronectin, to form granulation tissue[16–18]. The final stage is remodelling, which can last for several years. The formation of new capillaries slows, facilitating maturation of blood vessels in the wound. Type III collagen is replaced by type I collagen in the extracellular matrix to create a denser matrix with a higher tensile strength. The differentiation of fibroblasts into myofibroblasts causes the wound to physically contract. However, owing to differences in collagen type, new tissue after healing does not fully regain its original strength[16–18].
Causes of delayed wound healing
Delayed wound healing can be caused by local and/or systemic factors. Local factors in the wound site include oxygen deficiency (causing chronic hypoxia), excessive exudate (causing maceration) or insufficient exudate (leading to desiccation), local infection, foreign bodies intensifying the inflammatory response, repetitive trauma, pressure/shear, and impaired vascular supply to the injury area[16,19].
Systemic factors that delay the healing process include the following[16,19]:
- Advanced age;
- Psychological stress;
- Ischemia;
- Chronic diseases such as diabetes mellitus;
- Cardiac, renal or hepatic failure;
- Obesity;
- High alcohol consumption;
- Smoking;
- Sex hormones.
Oestrogen insufficiency, for instance in postmenopausal women, is known to impair all stages of wound repair process, especially inflammation and regranulation, with improved wound healing being a potential benefit of hormone replacement therapy. Androgens can repress cutaneous repair in both acute and chronic wounds, retarding the healing process and increasing inflammation[20].
The process can also be delayed in people with immunocompromised conditions, such as acquired immunodeficiency syndrome, cancer and malnutrition, with deficiencies in protein, carbohydrates, amino acids, vitamins A, C and E, zinc, iron, magnesium all having an effect[16,19,21]. Certain medicines can also delay the process, such as glucocorticoid steroids, chemotherapeutic drugs and non-steroidal anti-inflammatory drugs[19,21].
The most common causes of delayed chronic wound healing are infection and biofilm formation: biofilms are microscopically identifiable in up to 60% of chronic and recurrent wounds, leading to significant morbidity and mortality and an escalated healthcare cost[5,22,23].
A wound is considered infected when there are sufficiently large numbers of microbes presenting in wound environment or sufficient virulence to raise either a local or systemic immune response.
The wound-infection continuum has three stages: contamination, colonisation and infection. In the contamination phase, micro-organisms are unlikely to replicate because of an unfavourable environment. Colonisation happens when microbes successfully multiply, but not in sufficient levels to destroy host defences. However, the accelerated loads and persistence of microbes in wound environments may prolong the inflammatory phase and delay wound healing. When bacteria invade deeper into the wound bed and proliferate speedily, they can provoke an immune reaction and initiate local infection. As pathogens proliferate beyond the boundaries of the wound, infection may spread into deeper tissues, adjacent tissues, fascia, muscle or local organs. Eventually, systemic infection, such as sepsis, can occur when microbes invade into the body via vascular vessels or lymphatic systems, affecting the entirety of the body[24,25].
Biofilm is an extracellular polymeric substance produced by bacteria that acts as a physical barrier, enveloping bacteria and protecting them from host defences and antimicrobial agents. Several pathogens isolated from chronic wounds are typically capable of forming biofilms, such as Staphylococcus aureus and Pseudomonas spp[5,23,24,26]. Biofilms persisting within chronic wounds can continuously stimulate host immunity, resulting in the prolonged release of nitric oxide, pro-inflammatory cytokines such as interleukin-1 and TNF-α, and free radicals, and activation of immune complexes and complement, causing the healing process to fail and convert to a chronic state[23,27]. Sustained inflammatory reactions also trigger an escalated level of matrix metalloproteases, which can disrupt the extracellular matrix[16].
Most of the time, wound infection is diagnosed via visual inspection based on clinical signs and symptoms, including the classic signs of heat, pain, swelling, suppuration, erythema and fever. Typical characteristics of an acute infected wound are pain, erythema, swelling, purulent drainage, heat and malodour. In addition, a chronic wound may display signs of delayed healing, wound breakdown, friable granulation, epithelial bridging and pocketing in granulation tissue, increasing pain and serious odour.
Microbiological analysis of a specimen from wound cultures (using tissue biopsy or wound swab, pus collection or debrided viable tissue) is performed to identify causative microorganisms and guide the choice of antimicrobial therapy. Traditional diagnostics can be time consuming, and some organisms can be difficult to culture, so molecular techniques including DNA sequencing may help with characterising genetic markers[25]. Other laboratory markers, such as C-reactive protein, have also been used as markers and imaging techniques, such as CT scanning and autofluorescence imaging, may help with real-time diagnosis[25,28].
Chronic wound infection management
In clinical practice, the evaluation and identification of underlying conditions that affect wound healing are vital to optimising wound care. Accurate assessment of causes and comorbidities will inform the best course of treatment, such as compression therapy for venous leg ulcers or offloading (relief of pressure points) for people with diabetic foot ulcers[29]. The underlying pathologies of wounds are numerous and failure to address them can lead to a failure in healing[2,29].
Once any underlying conditions are identified, the wound bed should be prepared to optimise the chance of healing. A wound hygiene approach should be considered; its core principle is to remove or minimise unwanted materials, such as biofilm, devitalised tissue and foreign debris, from the wound bed to kickstart the healing process[30]. A holistic patient and wound assessment will ensure wound pathology and wound biofilm are managed simultaneously[30]. The TIME framework (tissue, infection/inflammation, moisture balance, edges) is a systematic approach to wound management[31]. Wound-bed preparation and the TIME approach should be used alongside a holistic assessment of other patient factors such as pain, nutrition and hydration[2].
Use of antimicrobials and challenges of antimicrobial resistance
Effective management of infection in chronic wounds involves the removal of necrotic tissue, debris and biofilms using debridement plus the appropriate use of antimicrobials (including topical antiseptics and systemic antibiotics)[1,32].
Antiseptics
Antiseptics have a broad spectrum of bactericidal activity and are used externally for the purposes of eliminating bacterial colonisation, preventing infection, and potentially stimulating wound healing. They are less likely to cause antimicrobial resistance (AMR) than antibiotics and inhibit the development of microbes by disrupting cell walls and cytoplasmic membranes, denaturing proteins, and damaging bacterial DNA and RNA[5,23,33,34]. An ideal antiseptic agent should have broad-spectrum activity, a fast onset of action, long-lasting activity, be safe for healthy surrounding tissue, possess minimal allergenicity, be stable in blood and tissue protein, persistently remain within the wound bed, and potentially be active against biofilms[23,35]. Antiseptics, antimicrobial washes or surfactants can be used to clean the wound and peri-wound skin and prepare the wound bed for debridement[30].
A variety of antiseptic agents are used in clinical practice[23,34]:
- Anilides (triclocarban);
- Bisphenols (triclosan;)
- Alcohols (ethanol, isopropanol);
- Biguanides (chlorhexidine, polyhexanide);
- Halogen releasing compounds (polyvinyl pyrrolidone-iodine, cadexomer iodine);
- Heavy metal compounds (silver, mercury derivatives);
- Quaternary ammonium compounds (cetrimide, benzalkonium salts).
Antiseptics can also be used as an adjunct to other therapies (e.g. negative pressure therapies) in treating complicated wound types (e.g. diabetic foot ulcers, venous leg ulcers and sternal wounds)[36].
Systematic antibiotics
All open wounds will be colonised with bacteria, but antibiotic therapy is only required for those that are clinically infected[37]. Systemic antibiotic therapy should only be considered for the treatment of cellulitis, osteomyelitis, sepsis, lymphangitis, abscess, and invasive tissue infection. Inappropriate use of systemic antibiotics may increase the risk of side effects and contributes to emergence of AMR[5]. The choice of initial therapy and the duration is frequently empirical and should take into account the type of wound, severity of infection, suspected pathogens and local AMR[38]. With severe infections, broad-spectrum antibiotics should be used against both gram-positive and gram-negative organisms, while a relatively narrow spectrum agent is enough for most mild and many moderate infections[5].
A systematic review assessed the clinical and cost-effective efficacy of systemic and topical antibiotic agents in the treatment of chronic skin wounds. The authors of the review suggested that there was insufficient evidence to support any ‘routine’ use of systemic antibiotics in specific chronic wounds[39].
Appropriate and judicious use of antimicrobials must be considered when managing wounds. The use of topical antibiotics is not recommended for eliminating bacterial colonisation or wound infections because of their limited effectiveness, high risk of resistance and potential to cause contact allergy[5,35].
AMR occurs when microorganisms naturally evolve in ways that cause medicines used to treat infections to become ineffective, and these micro-organisms become resistant to most[40,41]. The misuse and overuse of antibiotics is a major cause of the emergence of AMR, via four main mechanisms[42]:
- Altering the target of drug;
- Enzymatic inactivation of antimicrobial compounds;
- Reduced permeability of the bacterial membrane;
- Increasing efflux of antibiotics.
Moreover, the multicellular nature of biofilm matrix is likely to give extra protection to bacteria communities, makes them resistant to antibiotics. There are several proposed mechanisms for AMR related to biofilm: the alteration of chemical environment within biofilm, slow or inadequate diffusion of the antibiotics into the biofilm, and a differentiated biofilm subpopulation[43].
Topical antimicrobial use plays an important role when the wound is clinically infected or there is a suspected biofilm. The British Society for Antimicrobial Chemotherapy and European Wound Management Association position paper highlighted antimicrobial stewardship (AMS) — a set of strategies to improve the appropriateness and minimise the adverse effects of antibiotic use — as being central to wound care treatment to improving patient outcomes, reducing microbial resistance and decreasing the spread of infections caused by multidrug-resistant organisms[37]. Effective AMS avoids the use of antimicrobial therapy when not indicated while enabling the prescribing of appropriate antimicrobial interventions when they are indicated to treat infection.
The UK government has outlined a 20-year vision for reducing AMR, proposing a lower burden of infection through better treatment of resistant infections[44]. This includes the optimal use of antimicrobials and good stewardship across all sectors and appropriate use of new diagnostics, therapies, vaccines and interventions in use, combined with a full AMR research and development pipeline for antimicrobials, alternatives, diagnostics, vaccines and infection prevention across all sectors.
The use of alternatives to traditional antibiotic therapy is of huge interest for combating increasing AMR, including bacteriophage therapy, phage-encoded products, monoclonal antibodies and immunotherapy[45]. Among these, endolysins — phage-encoded peptidoglycan hydrolases selectively targeting bacterial taxa — have been identified as promising antimicrobial agents because of their ability to kill antimicrobial-resistant bacteria and lack of reported resistance However, challenges restrict the widespread use of endolysin therapy, such as limited drug-delivery methods, their specificity to particular bacteria types, and bioavailability via IV administration[46,47].
Debridement
Debridement is the physical removal of biofilm, devitalised tissue, debris and organic matter and is a crucial component of wound care. The presence of non-viable tissue in the wound bed prevents the formation of granulation tissue and delays the wound healing process. The removal of non-viable tissue encourages wound healing. The type of tissue found in the wound bed (e.g. whether necrotic or sloughy) will determine whether debridement is required. Factors such as bioburden, wound edges and the condition of peri-wound skin can also influence whether debridement is required[48]. A range of techniques can be used, dependent on the clinician’s ability level: these include autolytic, larval, mechanical, sharp and surgical methods[49,50].
Wound dressings
The concept of moist wound healing is not new and can lead to healing up to 2–3 times quicker than that of dry wound healing[51,52]. Wound dressings such as cotton wool, gauze, plasters, bandages, tulle or lint should not be used, as they do not promote a moist wound healing environment, require excessive changes, and can cause skin damage and pain during dressing changes. They have therefore been replaced by newer types of wound dressings that can play a role in autolysis and debridement, maintain a relatively stable local temperature, keep the wound hydrated, promote wound repair and prevent bacterial infection[14,53,54].
Wound dressings should keep the wound free from infections, excessive slough, contaminants and poisons, keep the wound at the ideal temperature and optimum pH for healing, be permeable to water, but not microbes, come away from wound trauma during dressing changes, not be painful and be comfortable[55]. There are a variety of dressings available for managing chronic wounds, such as hydrogels, hydrocolloids, alginates, foams, and film dressings[56]. Dressings can also be used carriers for active agents including growth factors, antimicrobial agents, anti-inflammatory agents, monoterpenes, silver sulfadiazine or silver nanoparticles[57].
Potential factors that may influence dressing selection include:
- Type of wound/cause;
- Wound characteristics (e.g. granulating, epithelialising, sloughy, necrotic);
- Treatment goals (e.g. to manage exudate, manage infection risk);
- Anatomical location;
- Patient-related factors (e.g. pain levels, fragile skin, capacity to self-care);
- Cost.
Antimicrobial dressings impregnated with iodine, silver and honey are available[58]. They can be divided into two categories: those that release an antimicrobial into the wound and those that bind bacteria and remove them from the wound into the dressing. A more detailed overview can be found in a recent consensus document on wound care and dressing selection for pharmacists[57].
It is essential wound dressings do not inadvertently lead to moisture-associated skin damage — an umbrella term encapsulating incontinence-associated dermatitis, intertriginous dermatitis (or intertrigo), peri-wound maceration and peristomal dermatitis. Practitioners should ensure the dressing can manage any exudate and protects the peri-wound area. Skin barriers can be used to protect the peri-wound area and prevent skin damage[59].
Negative-pressure wound therapy
Widely used to aid the healing of acute, chronic and traumatic wounds, negative-pressure wound therapy (NPWT) removes interstitial fluid/oedema and excessive exudate, provides a moist environment, improves blood flow and tissue perfusion, and stimulates angiogenesis and granulation tissue formation[4,60]. Results from several studies have demonstrated the selective effect of NPWT in eliminating non-fermentative gram-negative bacilli in wounds[36]. Additionally, NPWT can be combined with additional topical antimicrobial solutions, reducing bacteria load, stimulating wound closure and decreasing wound size faster than conventional NPWT[36,61,62].
Hyperbaric oxygen therapy
Hyperbaric oxygen therapy was first proposed as an additional treatment for chronic wounds in the mid-1960s. Treatment involves the intermittent exposure of the body within a large chamber to 100% oxygen at a pressure between 2.0 and 2.5 atmosphere absolute, leading to an increase in oxygen levels within haemoglobin and elevating oxygen tissue tension at the wound site[63]. A Cochrane review published in 2015 reported a significant improvement in the healing of diabetes ulcers in the short term when treated with hyperbaric oxygen therapy. However, further high-quality studies are needed before clinical benefits can be proven[64].
Electrical stimulation
For chronic wound healing, electrical stimulation is the most frequently studied biophysical therapy[4]. It uses direct current, alternative current, and pulsed current. Electrical stimulation has been shown to benefit every stage of the wound-healing process, both at cellular and systemic levels. During the inflammation phase, electrical stimulation promotes vasodilation and increases the permeability of blood vessels, thereby facilitating cellular movement to the wound site and so promoting a shorter inflammatory response.
Studies have reported an inhibition in bacterial proliferation after electric stimulation. In the proliferation phase, electrical stimulation raises the migration, proliferation and differentiation of endothelial cells, keratinocytes, myofibroblasts and fibroblasts. At the systemic level, it promotes revascularisation, angiogenesis, collagen matrix organisation, wound contraction, and re-epithelialisation. Ultimately, electrical stimulation promotes the contractility of myofibroblast and converts type III collagen into type I, along with rearranging collagen fibres to optimise the scar’s tensile strength[8,65,66].
A prospective clinical study conducted across the UK suggested that using an externally applied electroceutical device, combined with compression bandaging and dressings, was a cost-effective treatment for venous leg ulcers, compared with conventional treatments[67].
Low-frequency ultrasound
Low-frequency ultrasound has been used as an adjunct treatment for chronic wounds. It has a debriding effect, removing debris and necrotic tissue (primarily via cavitational and acoustic streaming phenomena). Ultrasound is also reported to disrupt biofilm in vitro, thus increasing the sensitivity of bacteria to antimicrobials[68]. It is proposed to be effective in stimulating collagen synthesis, increasing angiogenesis, diminishing the inflammatory phase as well as promoting cellular proliferation[69]. Several clinical studies have shown a reduction in wound size when wounds are treated with low-frequency ultrasound therapy[70–72].
Extracorporeal shock wave therapy
Extracorporeal shock wave therapy (ESWT) has been proposed to aid wound healing by transmitting acoustic pulsed energy to tissues. ESWT seems to promote angiogenesis, stimulate circulation, reduce anti-inflammatory response, and upregulate cytokine and growth-factor reactions[4]. A clinical trial demonstrated the feasibility and tolerability of ESWT in wounds with different aetiologies[73]. Furthermore, a review concluded that ESWT brings more benefits for patients with diabetic foot ulcers than hyperbaric oxygen therapy, based on increased angiogenesis, tissue perfusion and cellular reactions with reduced cell apoptosis, as well as a higher ulcer healing rate[74].
Advanced therapies
More recent developments include introducing nanomedicine to wound-healing approaches. It has been used to achieve controlled delivery, stimulate chronic wound healing and control microbial infections[14,75]. Nanotechnology-based wound dressings like nanogels and nanofibers offer a larger surface area and greater porosity, potentially enhancing absorption of wound exudate. They can also facilitate collagen synthesis and ultimately re-epithelisation through supporting the migration and proliferation of fibroblasts and keratinocytes.
Nanomedicines also seem to aid healing through molecular and cellular pathways[75]. For example, a methacrylated gelatin (MeGel)/poly(L-lactic acid) hybrid nanofiber synthesised has been reported to stimulate the recruitment and proliferation of human dermal fibroblasts, thereby promoting wound healing[76]. Nanoparticles can not only act as carriers of antimicrobial agents, they can also have an intrinsic antimicrobial effect[75,77,78]. In 2021, Qiu et al. successfully developed an antibacterial photodynamic gold nanoparticle (AP-AuNPs) that demonstrated antibacterial effects on both Gram-negative Escherichia coli and Gram-positive Staphylococcus aureus, as well as potentially inhibiting biofilm formation in vitro[79].
Growth factors
Growth factors such as vascular endothelial growth factor (VEGF), platelet-derived growth factor (PDGF), and fibroblast growth factor (FGF) are down-regulated in chronic wounds, suggesting that topical administration of growth factors and cytokines could improve wound healing[80].
Growth factors can improve wound repair through several mechanisms[81]:
- By acting as chemo-attractants to promote the recruitment of inflammatory cells and fibroblasts into the wound site;
- By acting as mitogens to stimulate cellular proliferation;
- By stimulating angiogenesis and regeneration of blood vessels;
- By facilitating the synthesis of extracellular matrix;
- By affecting the cytokines and growth-factor-regulated synthesis of surrounding tissues.
Growth factors that have been studied in wound healing are EGF, VEGF, FGF, PDGF, transforming growth factor-beta 1 (TGF-β1) and granulocyte-macrophage colony stimulating factor[82]. Becaplermin (rhPDGF-BB) was the first growth-factor therapy approved by the US Food and Drug Administration, after it demonstrated effectiveness in treating complex wounds when combined with standard wound care[80]. A systematic review and meta-analysis indicated that growth factors were effective in healing venous stasis ulcers, increasing wound healing by 48.8% compared with placebo and showing no difference in adverse effects compared with controls[83].
Stem-cell therapies
Stem cells may have certain advantages in wound healing because of their ability to differentiate into specialised cells and secrete numerous mediators including cytokines, chemokines, and growth factors[84,85]. This makes them a promising approach for treating chronic wounds.
Mesenchymal stem cells can be extracted from bone marrow, adipose tissue, umbilical cord blood, nerve tissue, or dermis and used both systemically and locally[86]. They release growth factors that stimulate blood-vessel and granulation tissue formation, fibroblast and keratinocyte migration, collagen synthesis, and fibroblast activation, increase re-epithelialisation, exert immunomodulatory properties, regulate inflammatory responses, and display antibacterial activities[85,87–89].
Many studies have investigated the efficacy of stem-cell therapies for a variety of wounds, including burns, non-healing ulcers, and critical limb ischemia[90–96]. A systematic review published in 2020 that investigated the clinical application of stem-cell therapy for the treatment of chronic wounds showed the potential of a variety of stem cells in the restoration of impaired wound healing, both in vitro and in vivo, despite the clinical evidence being very limited. As the recorded studies were on case-by-case basis, there is a lack of comprehensive guidelines for the use of stem cells in different wounds[97].
Auto-transplantation of adipose tissue-derived mesenchymal stromal cells has been proposed as a safe, alternative method to treat chronic venous ulcers[96]. Bioscaffold matrices comprising hyaluronic acid, collagen or other bio-polymeric materials have increasingly been applied for stem-cell transplantation. These matrices not only provide wound coverage, but also offer protection for stem cells and controlled delivery[86].
Bioengineered tissues and skin equivalents
Skin equivalents are polymeric biomaterials increasingly adopted for both acute and non-healing ulcers, such as venous ulcers, diabetic foot ulcers or pressure ulcers, to temporarily or permanently substitute the structure and function of human skin. Skin substitutes are designed to increase wound healing, provide a physical barrier that protects the wound from trauma or bacteria, provide a moist environment for the repair process, replace impaired skin components and decrease morbidity from more invasive treatments like skin grafting[98,99].
They can usually be classified as one of three major types: dermal replacement, epidermal replacement, and dermal/epidermal replacement[98]. Epidermal replacements (substitutes) comprising isolated autogeneous keratinocytes cultured on top of fibroblasts include Myskin (Regenerys), Laserskin (Fidia Advanced Biopolymers) and Epicel (Genzyme Tissue Repair Corporation). Dermal replacements include Dermagraft (Smith and Nephew) and Transcyte (Shire Regenerative Medicine)[98].
Epidermal/dermal skin replacements (also called composite skin substitutes) contain both epidermal and dermal layers that mimic the histological structure of original skin. The bi-layered bioengineering skin Apligraf (Organogenesis) was the first living skin equivalent for the management of complex chronic wounds like diabetic foot ulcers and venous leg ulcers. It is made up of a dermal layer of human fibroblasts embedded in a bovine type I collagen matrix and an epidermal layer generated by human keratinocytes[100]. Some other commercial products of composite substitutes are OrCel (Forticell Bioscience) and PermaDerm (Regenicin)[98]. In general, the current high cost of such dressings and limited evidence on effectiveness restricts them from being widely adopted[101]. Recently, technologies such as electrospinning or 3D-printing have been used to fabricate skin substitutes. Electrospinning can create nanofibers with high oxygen permeability, variable porosity, a large, exposed surface area and a morphology similar to the extracellular matrix, making them interesting candidates for skin substitutes[102,103].
Conclusion and future perspectives
The National Wound Care Strategy Programme, which was implemented by NHS England in 2018, has made progress in reducing unwanted variation in care and addressing suboptimal wound care.
Through its workstreams, the involvement of stakeholders, patients and carers, and the publication of the core capabilities for educating a multi-professional workforce, wound care has become a national priority. There are still many challenges in the management of chronic wounds — the complexity of wound environment, limited knowledge of the biological, biochemical, and immunological healing processes, and the increasing complexity of disease pathophysiology that comes with ageing populations.
The development of standardised and clinically relevant testing for wound dressings, along with high-quality clinical trials, would enable useful comparisons of treatments. The whole episode of care should be considered in assessments of the cost-effectiveness of different dressings and devices, rather the simple cost of the individual entity. All these complex concerns restrict success in wound management, which in turn negatively impacts the quality of life of the patients and places a burden on global healthcare systems[75,104]. Organisations and healthcare providers should share best practice and education of healthcare professionals is needed to get the best outcomes for patients with preventable chronic wounds.
- 1Pang C, Ibrahim A, Bulstrode NW, et al. An overview of the therapeutic potential of regenerative medicine in cutaneous wound healing. Int Wound J. 2017;14:450–9. doi:10.1111/iwj.12735
- 2Atkin L. Chronic wounds: the challenges of appropriate management. Br J Community Nurs. 2019;24:S26–32. doi:10.12968/bjcn.2019.24.sup9.s26
- 3Menke NB, Ward KR, Witten TM, et al. Impaired wound healing. Clinics in Dermatology. 2007;25:19–25. doi:10.1016/j.clindermatol.2006.12.005
- 4Frykberg RG, Banks J. Challenges in the Treatment of Chronic Wounds. Advances in Wound Care. 2015;4:560–82. doi:10.1089/wound.2015.0635
- 5Siddiqui AR, Bernstein JM. Chronic wound infection: Facts and controversies. Clinics in Dermatology. 2010;28:519–26. doi:10.1016/j.clindermatol.2010.03.009
- 6Sen CK, Gordillo GM, Roy S, et al. Human skin wounds: A major and snowballing threat to public health and the economy. Wound Repair and Regeneration. 2009;17:763–71. doi:10.1111/j.1524-475x.2009.00543.x
- 7Guest JF, Fuller GW, Vowden P. Cohort study evaluating the burden of wounds to the UK’s National Health Service in 2017/2018: update from 2012/2013. BMJ Open. 2020;10:e045253. doi:10.1136/bmjopen-2020-045253
- 8Hunckler J, de Mel A. A current affair: electrotherapy in wound healing. JMDH. 2017;Volume 10:179–94. doi:10.2147/jmdh.s127207
- 9Kwon DS, Gao X, Liu YB, et al. Treatment with bone marrow-derived stromal cells accelerates wound healing in diabetic rats. Int Wound Journal. 2008;5:453–63. doi:10.1111/j.1742-481x.2007.00408.x
- 10Gosain A, DiPietro LA. Aging and Wound Healing. World Journal of Surgery. 2004;28:321–6. doi:10.1007/s00268-003-7397-6
- 11Enoch S, Leaper DJ. Basic science of wound healing. Surgery (Oxford). 2008;26:31–7. doi:10.1016/j.mpsur.2007.11.005
- 12Bhagavan N, Ha C-E. Biochemistry of hemostasis. In: Essentials of Medical Biochemistry . Academic Press 2015. 752.
- 13Gale AJ. Continuing Education Course #2: Current Understanding of Hemostasis. Toxicol Pathol. 2010;39:273–80. doi:10.1177/0192623310389474
- 14Maaz Arif M, Khan SM, Gull N, et al. Polymer-based biomaterials for chronic wound management: Promises and challenges. International Journal of Pharmaceutics. 2021;598:120270. doi:10.1016/j.ijpharm.2021.120270
- 15Pratt J, West G. Pressure therapy: history and rationale. In: Pressure garments: A manual on their design and fabrication. Butterworth–Heinemann 1995. 146.
- 16Guo S, DiPietro LA. Factors Affecting Wound Healing. J Dent Res. 2010;89:219–29. doi:10.1177/0022034509359125
- 17Broughton G II, Janis JE, Attinger CE. The Basic Science of Wound Healing. Plastic and Reconstructive Surgery. 2006;117:12S-34S. doi:10.1097/01.prs.0000225430.42531.c2
- 18Landén NX, Li D, Ståhle M. Transition from inflammation to proliferation: a critical step during wound healing. Cell. Mol. Life Sci. 2016;73:3861–85. doi:10.1007/s00018-016-2268-0
- 19Khalil H, Cullen M, Chambers H, et al. Elements affecting wound healing time: An evidence based analysis. Wound Rep and Reg. 2015;23:550–6. doi:10.1111/wrr.12307
- 20Horng H-C, Chang W-H, Yeh C-C, et al. Estrogen Effects on Wound Healing. IJMS. 2017;18:2325. doi:10.3390/ijms18112325
- 21Phillips SJ. Physiology of Wound Healing and Surgical Wound Care. ASAIO Journal. 2000.https://journals.lww.com/asaiojournal/fulltext/2000/11000/physiology_of_wound_healing_and_surgical_wound.29.aspx (accessed Jul 2022).
- 22Leaper D, Assadian O, Edmiston CE. Approach to chronic wound infections. Br J Dermatol. 2015;173:351–8. doi:10.1111/bjd.13677
- 23James GA, Swogger E, Wolcott R, et al. Biofilms in chronic wounds. Wound Repair and Regeneration. 2008;16:37–44. doi:10.1111/j.1524-475x.2007.00321.x
- 24Zhao G, Usui ML, Lippman SI, et al. Biofilms and Inflammation in Chronic Wounds. Advances in Wound Care. 2013;2:389–99. doi:10.1089/wound.2012.0381
- 25Li S, Renick P, Senkowsky J, et al. Diagnostics for Wound Infections. Advances in Wound Care. 2021;10:317–27. doi:10.1089/wound.2019.1103
- 26Wolcott RD, Rhoads DD. A study of biofilm-based wound management in subjects with critical limb ischaemia. J Wound Care. 2008;17:145–55. doi:10.12968/jowc.2008.17.4.28835
- 27Wolcott RD, Rhoads DD, Dowd SE. Biofilms and chronic wound inflammation. J Wound Care. 2008;17:333–41. doi:10.12968/jowc.2008.17.8.30796
- 28Wound Infection In Clinical Practice: Principles of best practice. Wounds International. 2022.https://www.woundsinternational.com/resources/details/wound-infection-in-clinical-practice-principles-of-best-practice (accessed Jul 2022).
- 29Darwin E, Tomic-Canic M. Healing Chronic Wounds: Current Challenges and Potential Solutions. Curr Derm Rep. 2018;7:296–302. doi:10.1007/s13671-018-0239-4
- 30Murphy C, Atkin L, Swanson T, et al. Defying hard-to-heal wounds with an early antibiofilm intervention strategy: wound hygiene. J Wound Care. 2020;29:S1–26. doi:10.12968/jowc.2020.29.sup3b.s1
- 31Schultz GS, Sibbald RG, Falanga V, et al. Wound bed preparation: a systematic approach to wound management. Wound Repair Regen. 2003;11:S1–28. doi:10.1046/j.1524-475x.11.s2.1.x
- 32Davis SC, Martinez L, Kirsner R. The diabetic foot: The importance of biofilms and wound bed preparation. Curr Diab Rep. 2006;6:439–45. doi:10.1007/s11892-006-0076-x
- 33Landis SJ. Chronic Wound Infection and Antimicrobial Use. Advances in Skin & Wound Care. 2008;21:531–40. doi:10.1097/01.asw.0000323578.87700.a5
- 34McDonnell G, Russell AD. Antiseptics and Disinfectants: Activity, Action, and Resistance. Clin Microbiol Rev. 1999;12:147–79. doi:10.1128/cmr.12.1.147
- 35Daeschlein G. Antimicrobial and antiseptic strategies in wound management. Int Wound J. 2013;10:9–14. doi:10.1111/iwj.12175
- 36Glass GE, Murphy GRF, Nanchahal J. Does negative-pressure wound therapy influence subjacent bacterial growth? A systematic review. Journal of Plastic, Reconstructive & Aesthetic Surgery. 2017;70:1028–37. doi:10.1016/j.bjps.2017.05.027
- 37Lipsky BA, Dryden M, Gottrup F, et al. Antimicrobial stewardship in wound care: a Position Paper from the British Society for Antimicrobial Chemotherapy and European Wound Management Association. J. Antimicrob. Chemother. 2016;71:3026–35. doi:10.1093/jac/dkw287
- 38Hernandez R. The use of systemic antibiotics in the treatment of chronic wounds. Dermatol Ther. 2006;19:326–37. doi:10.1111/j.1529-8019.2006.00091.x
- 39O’Meara S, Cullum N, Majid M, et al. Systematic reviews of wound care management: (3) antimicrobial agents for chronic wounds; (4) diabetic foot ulceration. Health Technol Assess. 2001;4. doi:10.3310/hta4210
- 40Antimicrobial Resistance. World Health Organization. 2021.https://www.who.int/news-room/fact-sheets/detail/antimicrobial-resistance (accessed Jul 2022).
- 41Edwards-Jones V. Antimicrobial resistance – challenges for the 21st century. Wounds International. 2018.https://www.woundsinternational.com/resources/details/antimicrobial-resistance-challenges-21st-century (accessed Jul 2022).
- 42Moo C-L, Yang S-K, Yusoff K, et al. Mechanisms of Antimicrobial Resistance (AMR) and Alternative Approaches to Overcome AMR. CDDT. 2020;17:430–47. doi:10.2174/1570163816666190304122219
- 43Sharma D, Misba L, Khan AU. Antibiotics versus biofilm: an emerging battleground in microbial communities. Antimicrob Resist Infect Control. 2019;8. doi:10.1186/s13756-019-0533-3
- 44Contained and Controlled: The UK’s 20-Year Vision for Antimicrobial Resistance. Gov.uk. 2019.https://www.gov.uk/government/publications/uk-20-year-vision-for-antimicrobial-resistance2019 (accessed Jul 2022).
- 45Chang RYK, Nang SC, Chan H-K, et al. Novel antimicrobial agents for combating antibiotic-resistant bacteria. Advanced Drug Delivery Reviews. 2022;187:114378. doi:10.1016/j.addr.2022.114378
- 46Murray E, Draper LA, Ross RP, et al. The Advantages and Challenges of Using Endolysins in a Clinical Setting. Viruses. 2021;13:680. doi:10.3390/v13040680
- 47Roach DR, Donovan DM. Antimicrobial bacteriophage-derived proteins and therapeutic applications. Bacteriophage. 2015;5:e1062590. doi:10.1080/21597081.2015.1062590
- 48Atkin L, Stephenson J, Parfitt G, et al. An investigation to assess ankle mobility in healthy individuals from the application of multi-component compression bandages and compression hosiery. J Foot Ankle Res. 2016;9. doi:10.1186/s13047-016-0151-8
- 49Moffatt C, Martin R, Smithdale R. Leg ulcer management. Blackwell Publishing 2007.
- 50Halim AS, Khoo TL, Mat Saad AZ. Wound bed preparation from a clinical perspective. Indian J Plast Surg. 2012;45:193–202. doi:10.4103/0970-0358.101277
- 51WINTER GD. Formation of the Scab and the Rate of Epithelization of Superficial Wounds in the Skin of the Young Domestic Pig. Nature. 1962;193:293–4. doi:10.1038/193293a0
- 52Swezey L. Moist wound healing. Wound Educators. 2014.https://woundeducators.com/wound-moisture-balance/ (accessed Jul 2022).
- 53Moore ZE, Webster J. Dressings and topical agents for preventing pressure ulcers. Cochrane Database of Systematic Reviews. 2018;2018. doi:10.1002/14651858.cd009362.pub3
- 54Shi C, Wang C, Liu H, et al. Selection of Appropriate Wound Dressing for Various Wounds. Front. Bioeng. Biotechnol. 2020;8. doi:10.3389/fbioe.2020.00182
- 55Wound management products and elasticated garments. British National Formulary . 2016.https://bnf.nice.org.uk/wound-management (accessed Jul 2022).
- 56Norman G, Westby MJ, Rithalia AD, et al. Dressings and topical agents for treating venous leg ulcers. Cochrane Database of Systematic Reviews. 2018;2018. doi:10.1002/14651858.cd012583.pub2
- 57Wound care and dressing selection for pharmacy teams. Wounds UK. 2021.https://www.wounds-uk.com/resources/details/wound-care-and-dressing-selection-pharmacy-teams (accessed Jul 2022).
- 58Mohseni M, Shamloo A, Aghababaie Z, et al. A comparative study of wound dressings loaded with silver sulfadiazine and silver nanoparticles: In vitro and in vivo evaluation. International Journal of Pharmaceutics. 2019;564:350–8. doi:10.1016/j.ijpharm.2019.04.068
- 59Woo KY, Beeckman D, Chakravarthy D. Management of Moisture-Associated Skin Damage: A Scoping Review. Adv Skin Wound Care. 2017;30:494–501. doi:10.1097/01.asw.0000525627.54569.da
- 60Streubel PN, Stinner DJ, Obremskey WT. Use of Negative-pressure Wound Therapy in Orthopaedic Trauma. Journal of the American Academy of Orthopaedic Surgeons. 2012;20:564–74. doi:10.5435/jaaos-20-09-564
- 61Giri P, Krishnaraj B, Chandra Sistla S, et al. Does negative pressure wound therapy with saline instillation improve wound healing compared to conventional negative pressure wound therapy? – A randomized controlled trial in patients with extremity ulcers. Annals of Medicine and Surgery. 2021;61:73–80. doi:10.1016/j.amsu.2020.12.015
- 62Gabriel A, Camardo M, O’Rorke E, et al. Effects of Negative-Pressure Wound Therapy With Instillation versus Standard of Care in Multiple Wound Types: Systematic Literature Review and Meta-Analysis. Plastic & Reconstructive Surgery. 2020;147:68S-76S. doi:10.1097/prs.0000000000007614
- 63Hajhosseini B, Kuehlmann BA, Bonham CA, et al. Hyperbaric Oxygen Therapy. Plastic and Reconstructive Surgery – Global Open. 2020;8:e3136. doi:10.1097/gox.0000000000003136
- 64Kranke P, Bennett MH, Martyn-St James M, et al. Hyperbaric oxygen therapy for chronic wounds. Cochrane Database of Systematic Reviews. 2015. doi:10.1002/14651858.cd004123.pub4
- 65Cheah YJ, Buyong MR, Mohd Yunus MH. Wound Healing with Electrical Stimulation Technologies: A Review. Polymers. 2021;13:3790. doi:10.3390/polym13213790
- 66Ud-Din S, Bayat A. Electrical Stimulation and Cutaneous Wound Healing: A Review of Clinical Evidence. Healthcare. 2014;2:445–67. doi:10.3390/healthcare2040445
- 67Guest JF, Ayoub N, Greaves T. Clinical outcomes and cost-effectiveness of an externally applied electroceutical device in managing venous leg ulcers in clinical practice in the UK. J Wound Care. 2015;24:572–80. doi:10.12968/jowc.2015.24.12.572
- 68Chang Y-JR, Perry J, Cross K. Low-Frequency Ultrasound Debridement in Chronic Wound Healing: A Systematic Review of Current Evidence. Plast Surg (Oakv). 2017;25:21–6. doi:10.1177/2292550317693813
- 69Breuing KH, Bayer L, Neuwalder J, et al. Early Experience Using Low-Frequency Ultrasound in Chronic Wounds. Annals of Plastic Surgery. 2005;55:183–7. doi:10.1097/01.sap.0000168695.20350.07
- 70Kavros SJ, Miller JL, Hanna SW. Treatment of Ischemic Wounds with Noncontact, Low-Frequency Ultrasound. Advances in Skin & Wound Care. 2007;20:221–6. doi:10.1097/01.asw.0000266660.88900.38
- 71Ennis WJ, Valdes W, Gainer M, et al. Evaluation of Clinical Effectiveness of MIST Ultrasound Therapy for the Healing of Chronic Wounds. Advances in Skin & Wound Care. 2006;19:437–46. doi:10.1097/00129334-200610000-00011
- 72Driver VR, Yao M, Miller CJ. Noncontact low-frequency ultrasound therapy in the treatment of chronic wounds: A meta-analysis. Wound Repair and Regeneration. 2011;19:475–80. doi:10.1111/j.1524-475x.2011.00701.x
- 73Schaden W, Thiele R, Kölpl C, et al. Shock Wave Therapy for Acute and Chronic Soft Tissue Wounds: A Feasibility Study. Journal of Surgical Research. 2007;143:1–12. doi:10.1016/j.jss.2007.01.009
- 74Wang C-J, Cheng J-H, Kuo Y-R, et al. Extracorporeal shockwave therapy in diabetic foot ulcers. International Journal of Surgery. 2015;24:207–9. doi:10.1016/j.ijsu.2015.06.024
- 75Sharifi S, Hajipour MJ, Gould L, et al. Nanomedicine in Healing Chronic Wounds: Opportunities and Challenges. Mol. Pharmaceutics. 2020;18:550–75. doi:10.1021/acs.molpharmaceut.0c00346
- 76Sun M, Chen S, Ling P, et al. Electrospun Methacrylated Gelatin/Poly(L-Lactic Acid) Nanofibrous Hydrogel Scaffolds for Potential Wound Dressing Application. Nanomaterials. 2021;12:6. doi:10.3390/nano12010006
- 77Liu Y, Shi L, Su L, et al. Nanotechnology-based antimicrobials and delivery systems for biofilm-infection control. Chem. Soc. Rev. 2019;48:428–46. doi:10.1039/c7cs00807d
- 78Gupta A, Mumtaz S, Li C-H, et al. Combatting antibiotic-resistant bacteria using nanomaterials. Chem. Soc. Rev. 2019;48:415–27. doi:10.1039/c7cs00748e
- 79Qiu L, Wang C, Lan M, et al. Antibacterial Photodynamic Gold Nanoparticles for Skin Infection. ACS Appl. Bio Mater. 2021;4:3124–32. doi:10.1021/acsabm.0c01505
- 80Yamakawa S, Hayashida K. Advances in surgical applications of growth factors for wound healing. Burns & Trauma. 2019;7. doi:10.1186/s41038-019-0148-1
- 81Greenhalgh DG. The Role of Growth Factors in Wound Healing. The Journal of Trauma: Injury, Infection, and Critical Care. 1996;41:159–67. doi:10.1097/00005373-199607000-00029
- 82Viaña‐Mendieta P, Sánchez ML, Benavides J. Rational selection of bioactive principles for wound healing applications: Growth factors and antioxidants. International Wound Journal. 2021;19:100–13. doi:10.1111/iwj.13602
- 83Lee Y, Lee M, Phillips S, et al. Growth factors for treating chronic venous leg ulcers: A systematic review and meta-analysis. Wound Repair Regen 2022;30:117–25. doi:10.1111/wrr.12982
- 84da Silva Meirelles L, Fontes AM, Covas DT, et al. Mechanisms involved in the therapeutic properties of mesenchymal stem cells. Cytokine & Growth Factor Reviews. 2009;20:419–27. doi:10.1016/j.cytogfr.2009.10.002
- 85Kanji S, Das H. Advances of Stem Cell Therapeutics in Cutaneous Wound Healing and Regeneration. Mediators of Inflammation. 2017;2017:1–14. doi:10.1155/2017/5217967
- 86Duscher D, Barrera J, Wong VW, et al. Stem Cells in Wound Healing: The Future of Regenerative Medicine? A Mini-Review. Gerontology. 2015;62:216–25. doi:10.1159/000381877
- 87Smith AN, Willis E, Chan VT, et al. Mesenchymal stem cells induce dermal fibroblast responses to injury. Experimental Cell Research. 2010;316:48–54. doi:10.1016/j.yexcr.2009.08.001
- 88Nie C, Yang D, Xu J, et al. Locally Administered Adipose-Derived Stem Cells Accelerate Wound Healing through Differentiation and Vasculogenesis. Cell Transplant. 2011;20:205–16. doi:10.3727/096368910×520065
- 89Kosaric N, Kiwanuka H, Gurtner GC. Stem cell therapies for wound healing. Expert Opinion on Biological Therapy. 2019;19:575–85. doi:10.1080/14712598.2019.1596257
- 90Kølle S-FT, Fischer-Nielsen A, Mathiasen AB, et al. Enrichment of autologous fat grafts with ex-vivo expanded adipose tissue-derived stem cells for graft survival: a randomised placebo-controlled trial. The Lancet. 2013;382:1113–20. doi:10.1016/s0140-6736(13)61410-5
- 91Ravari H, Hamidi-Almadari D, Salimifar M, et al. Treatment of non-healing wounds with autologous bone marrow cells, platelets, fibrin glue and collagen matrix. Cytotherapy. 2011;13:705–11. doi:10.3109/14653249.2011.553594
- 92Dash NR, Dash SN, Routray P, et al. Targeting Nonhealing Ulcers of Lower Extremity in Human Through Autologous Bone Marrow-Derived Mesenchymal Stem Cells. Rejuvenation Research. 2009;12:359–66. doi:10.1089/rej.2009.0872
- 93OTERO G, AGORIO C, SUJANOV A, et al. Autologous bone marrow–derived cells for venous leg ulcers treatment: a pilot study. Cytotherapy. 2019;21:189–99. doi:10.1016/j.jcyt.2019.01.002
- 94Kavala A, Turkyilmaz S. Autogenously derived regenerative cell therapy for venous leg ulcers. Arch Med Sci Atheroscler Dis. 2018;3:156–63. doi:10.5114/amsad.2018.81000
- 95Larsen L, Tchanque-Fossuo CN, Gorouhi F, et al. Combination therapy of autologous adipose mesenchymal stem cell-enriched, high-density lipoaspirate and topical timolol for healing chronic wounds. J Tissue Eng Regen Med. 2017;12:186–90. doi:10.1002/term.2390
- 96Masłowski L, Paprocka M, Czyżewska-Buczyńska A, et al. Autotransplantation of the Adipose Tissue-Derived Mesenchymal Stromal Cells in Therapy of Venous Stasis Ulcers. Arch. Immunol. Ther. Exp. 2020;68. doi:10.1007/s00005-020-00571-9
- 97Raghuram A, Yu R, Lo A, et al. Role of stem cell therapies in treating chronic wounds: A systematic review. World J Stem Cells 2020;12:659–75. doi:10.4252/wjsc.v12.i7.659
- 98Vyas K, Vasconez H. Wound Healing: Biologics, Skin Substitutes, Biomembranes and Scaffolds. Healthcare. 2014;2:356–400. doi:10.3390/healthcare2030356
- 99Dai C, Shih S, Khachemoune A. Skin substitutes for acute and chronic wound healing: an updated review. Journal of Dermatological Treatment. 2020;31:639–48. doi:10.1080/09546634.2018.1530443
- 100Zaulyanov L, Kirsner RS. A review of a bi-layered living cell treatment (Apligraf�) in the treatment of venous leg ulcers and diabetic foot ulcers. Clinical Interventions in Aging. 2007;2:93–8. doi:10.2147/ciia.2007.2.1.93
- 101Han G, Ceilley R. Chronic Wound Healing: A Review of Current Management and Treatments. Adv Ther. 2017;34:599–610. doi:10.1007/s12325-017-0478-y
- 102Augustine R, Kalarikkal N, Thomas S. Advancement of wound care from grafts to bioengineered smart skin substitutes. Prog Biomater. 2014;3:103–13. doi:10.1007/s40204-014-0030-y
- 103Tavakoli S, Klar AS. Bioengineered Skin Substitutes: Advances and Future Trends. Applied Sciences. 2021;11:1493. doi:10.3390/app11041493
- 104Mahmoudi M, Gould L. <p>Opportunities and Challenges of the Management of Chronic Wounds: A Multidisciplinary Viewpoint</p> CWCMR. 2020;Volume 7:27–36. doi:10.2147/cwcmr.s260136